7-A NAE and White House Guidelines
As described in Chapter 1, “ERC Program Origins,” section 1-B(d), the impetus for the creation of the National Science Foundation’s landmark Engineering Research Centers (ERC) Program came from the highest reaches of the U.S. government, specifically from the White House Science Advisor, along with detailed guidance from the National Academy of Engineering (NAE). One of the main drivers of the Program was the perceived need to reconnect academic engineering with U.S. industry, a connection that had largely been lost in the years following World War II. An important component of this goal was to refocus the education of engineering students in a way that would benefit industry, ultimately strengthening U.S. industrial competitiveness.
7-A(a) Motivation: Educate Engineers To Be Engineers
In the postwar years, engineering faculty had pulled away from their traditional focus on making things—on developing the technologies leading to products and processes—and on working closely with industry to facilitate that activity, and had moved in the direction of more abstract, theoretical pursuits such as simulation and modeling. As described in Chapter 1, much of the motivation for this shift was the wish to elevate the status of engineering faculty on campus to be more on a par with that of scientists.
As a consequence, engineering faculty had less and less familiarity with industrial practice and had begun to impart an education to their students that resulted in graduates resembling their professors—i.e., science-oriented, reductionist theoreticians rather than engineers ready to practice engineering with an integrative systems perspective. This trend had become very noticeable to leaders in industry and to some in government; one of the primary aims of the ERC Program was to begin to reverse it. What was needed was clear to the proponents of this groundbreaking new program.
i. Produce Graduates Who Are More Effective in Industrial Practice
One of the basic challenges posed to the new centers by the NAE’s Guidelines for Engineering Research Centers was that they should “Increase the number of engineering graduates who can contribute innovatively to U.S. productivity.”[1] They would accomplish this by involving industry more directly in engineering education; by increasing their contact with industrial practitioners through internships and on-campus presence; by increasing students’ experience on research teams; and other means. As the summary of a symposium held at the National Academy of Engineering to introduce the first class of ERCs put it:
The changes in engineering education are likely to be substantial for participating students. [Speaker Roland Schmitt] pointed out that it has been difficult for a student to acquire both the needed scientific knowledge and the apprenticeship aspects of education. Unlike education in the sciences, it is rare for engineering graduate students to be trained in the type of facilities they will encounter in industry. And engineering is the only profession in which teachers are not, by and large, experienced practitioners. [Speaker Jerrier Haddad] believes that the ERCs will go a long way toward changing this situation. For one thing, the closer contact of academic researchers with industry problems and methods will make them better teachers. More fundamentally, however, participation of students in ERC research programs will be a form of interning. It will introduce the missing element of practice, conferring practical values, greater interest in the work, and stronger personal development as well.[2]
The thinking was that, if the ERCs could attract top students and impart to them “a broad understanding of what is needed to bring sophisticated products all the way from the laboratory to the market,” the graduates of the centers would become “a most effective form of advertisement for the cross-disciplinary approach to research.”
These were visionary views at the time. They flew in the face of decades of academic engineering culture and would have lasting repercussions throughout engineering education, not just at ERC host universities, but in colleges of engineering nationwide.
ii. Integrate Research into Education
Another basic challenge posed to ERCs by the NAE guidelines, in this case with respect to engineering education, was that they needed to “Firmly link research and education.”[3] The connection between cutting-edge engineering research and the classroom had become more tenuous over time. Textbooks tended to remain static and “classic,” with only doctoral students being involved in serious research. Laboratory measurement and testing instrumentation as well as benchtop fabrication facilities had generally been neglected in favor of the latest computation equipment for conducting simulation and modeling. The Guidelines noted that the new centers “…can be especially effective in amplifying their impact on undergraduate engineering students by developing educational materials such as textbooks and laboratory experiments.”[4] They further prescribed “explicit efforts to extend the educational impact of the work done within the Centers through codification of the new knowledge to introduce it into the classroom…”[5] The designers of the guidelines, who included leaders from industry, were anxious that engineering graduates have state- of-the-art knowledge derived from cutting-edge research and not drawn from out-of-date publications.
As a result, the first ERC Program Announcement repeated the NAE’s guidance in a requirement that proposals should “firmly link engineering education and research, key elements in improving U.S. industrial productivity.”[6]
7-A(b) Focus: Students Doing Research in Partnership with Industry Imparts Systems Understanding
“Shop” Shock Lynn Preston remembers her first introductory tour of the School of Engineering at Purdue University in 1985, shortly after the new ERC there was founded. She was guided through the laboratories and the work on computer integrated manufacturing. When she asked where the facilities were for students to “make things,” thinking about how they would construct testbeds, she was told that the “shop” had been moved to the basement. When she asked for a tour of the basement shop there was a look of shock on the face of her guide, but she insisted. Down they went, and there in the basement she was met by the person responsible for helping engineers understand how to use tools and make things, as opposed to just modeling them or designing them on a computer. Her goal was to join the skills inherent in modeling and theory with those inherent in making things through testbeds to produce more effective engineering graduates, so the “shop” was as important as the lab. |
One of the iconoclastic elements of the ERC concept from its inception was the notion of the integrated “systems” aspects of engineering practice and thus the need for this type of broader focus in academic engineering research and education alike that would better reflect the complexities of engineering decisions in industry. (For background on the systems approach, see Churchman, 1979.[7]) Therefore, one of the ERC “key features” described in the first Program Announcement was that ERCs should:
- Emphasize the systems aspects of engineering and help educate and train students in synthesizing, integrating, and managing engineering systems.[8]
The Program’s developers recognized that, in order for students to gain an understanding of these aspects of real-world engineering, a necessary element of engineering education would be direct exposure to industry practice and practitioners—not only through internships and summer employment, but also through involvement of industry representatives in the planning of ERC education programs and on center research teams. Further, the center’s students—at all levels—would need to be included on those research teams. In that way they would gain not only a perspective on industrial practices and methods, but also experience in working on teams, such as those they would encounter in industry.
To that end, another key feature required of ERCs in the first Program Announcement was that they:
- Include a significant component involving both graduate and undergraduate students in the research activities of the Center throughout the academic year. Student participation in the activities of the Center would expose future engineers to many aspects of engineering rather than one specific field and better prepare them for the systems nature of engineering practice.[9]
So central to the functioning of ERCs was the systems concept that soon after their founding the NSF commissioned a study by the National Research Council (NRC) specifically on this topic. The study committee reported on their findings in 1986.[10] They described the goal for education as follows:
Students should learn how systems are designed, manufactured, and supported in the field, and what the goals of good engineering are. Such understanding is best obtained by hand-on experimentation in system design and development, and through exposure to industry personnel and methods of practice.[11]
This new (or rather, renewed) way of looking at the aims of engineering education took hold gradually in American colleges of engineering, radiating outward from ERC host institutions. Its spread was aided by continuing “proselytizing” by the NAE/NRC (see linked file “The Systems Context,” for example), by Congressional support for the ERC Program, by many teams of faculty struggling to create a systems vision for their ERC, and by industrial enthusiasm for the ERCs and their graduates as a new and valuable source of technology and engineering employees, as the first generation of centers got fully underway.
7-B Start-up: 1985–1990 (Gen-1)
A total of 21 ERCs were awarded during the Gen-1 phase of the program. As was described in Section 7-A, a strong emphasis was placed on revamping engineering education to ensure that graduates were better prepared for productive practice in industry. Education was one leg of the “three-legged stool” of ERC research, education, and industrial collaboration. Nam Suh, the Assistant Director for Engineering during this start-up period, advised the ERC Program leaders to enable the ERCs to experiment with new approaches for engineering education that would fulfill the Program’s goals and not to rely on the status quo in education at NSF that had been laid down by scientists who knew little about engineering or engineering practice.
Initially the primary emphasis was on research and its impact on new technology; but as experience with the ERCs evolved during the first few years of the Program, it became increasingly clear that both NSF and, more importantly, industry saw the graduates as an equally important output of the centers. In contrast to the occasional major discoveries and major technological innovations, graduates were constantly being produced and were adding value to their industrial employers on a daily basis as innovative team members who were ready to hit the ground running as new hires.
The first ERC Program Announcement listed as one of the three “defining characteristics” of ERCs that they “must contribute to the increased effectiveness of all levels of engineering education.” Among their key features, ERCs would “Emphasize the systems aspects of engineering and help educate and train students in synthesizing, integrating, and managing engineering systems”; and they would “Include a significant education component, involving both graduate and undergraduate students in research…[and involve] codification of new knowledge generated at the center.. .”[12] Education was listed among the six general criteria used in the evaluation of ERC proposals, specifically through: “Integration of the Center into the education of engineers, both at the undergraduate and graduate level.”[13] Over time, education became an increasingly important focus of ERCs. Consequently, as a 1995 report on education in the ERCs noted, “The ERCs devote a large amount of time and resources to ‘spreading the culture’ through education, and to creating an environment conducive to the new type of education that ERCs try to impart to students.”[14]
This section focuses on the education programs and goals of the first five classes of ERCs awarded between 1985 and 1990, a time during which the earliest centers were breaking new ground but also had more time to develop their programs.
7-B(a) Undergraduate Education
From the beginning of the Program, all newly funded ERCs had in place education programs that focused on the research area of the center but emphasized the cross-disciplinary team approach to research, the industrial perspectives on design and manufacturing, and experience with engineering systems and testbeds. These were the new features of ERC education aimed at preparing the students to contribute quickly and productively to industry.
A novel feature of ERC education programs was their emphasis on undergraduate participation in research throughout the academic year. Recognizing that many graduates enter industrial practice with only the BS degree, ERCs were required to treat undergraduates not just as future graduate students, but as full-fledged members of the research teams and future professional engineers. Each of the earliest ERCs had at least one program through which undergraduates were engaged in center research. Undergraduates were routinely listed as co-authors of published ERC research papers. Most of the centers had (and still have today) at least 20 undergraduate participants; several had more than 50.
Most of the early ERC undergraduate research programs shared several main features, which are still largely seen today. Students come from any of the departments that relate to the center’s work and are selected from among the best students. Generally, each student worked with a designated faculty member and, under his or her supervision, conducted research with a graduate student mentor, receiving academic credit and in some cases a stipend for the work.
For example, Undergraduate Scholars at North Carolina State University’s Center for Advanced Electronic Materials Processing (Class of 1988) received a scholarship of $4,000 for the academic year and were offered an opportunity for summer appointment. They spent about 10 hours per week working on a designated research project during the academic year and submitted a written research report at the end of the year.
MIT’s Biotechnology Process Engineering Center (BPEC)[15] and the University of Maryland’s System Research Center (SRC), both members of the first ERC Class of 1985, were early leaders in undergraduate education and in ERC education generally. The MIT center began with 116 undergraduates enrolled in elective chemical engineering courses that included biotechnology process engineering content. Two interdisciplinary courses in biochemical processes and biotechnology were offered within the first two years. BPEC’s most innovative undergraduate program was the inclusion of its undergraduates in MIT’s Undergraduate Research Opportunity Program (UROP). As Center Director Daniel Wang noted in late 1986, UROP enabled undergraduates—even freshmen—to perform research in various disciplines throughout their educational careers. Five MIT departments, including not only engineering but also chemistry and biology, had undergraduates participating in UROP—by 1986, 53 of them in all, including several with double majors. “It is through this type of program that we feel undergraduates are introduced to the principles of biotechnology that they will one day need in graduate students or in industry,” Wang noted.[16]
Maryland’s SRC[17] also brought early ERC-inspired innovations to undergraduate education. In 1986 they offered four special-purpose courses in artificial intelligence (then a revolutionary field) to sophomores and juniors. They emphasized undergraduate research projects in which students were required to build “real systems.” They arranged weekly interdisciplinary systems colloquia for students and established procedures for co-advising and joint teaching across disciplinary lines. And they built an advanced design laboratory and insisted that students go through the entire design process, to increase their awareness of implementation and digital design issues. By 1990, the SRC had initiated Undergraduate Research Participation Awards.
The Optoelectronic Computing Systems Center at the University of Colorado (Class of 1987) had an Undergraduate Research Program that started small but was later expanded with a State grant to support 19 undergraduates.
Duke University’s Center for Emerging Cardiovascular Technologies (Class of 1987) established an Undergraduate Fellows Program, in which Fellows worked on research projects under the direction of ERC faculty advisors, committing an entire summer and two of their last three undergraduate semesters to ERC-related research. Industry members of this center formed an Educational Partners Program, with a pool of funds earmarked to support both undergraduate and graduate students with $5,000 annual stipends used to obtain industrially relevant research experience.[18]
The ERC for Intelligent Manufacturing Systems at Purdue University (Class of 1985) was also an early education innovator. Because Purdue was then the biggest producer of engineering graduates in the country, its size “offers both the challenge of overcoming massive inertia and the potential for enormous impact,” as its director, James Solberg, noted at the time. He expected that the ERC could influence the education of nearly 1000 students a year through a variety of new courses, some of them team-taught by industry representatives.[19] The latter courses led to a two-week summer institute starting in 1986, offered to faculty and students of 20 other universities interested in robotics and automation. Also, in 1986 the Purdue ERC began a Summer Undergraduate Research Interns (SURI) program, in which selected students joined an established research project team as full-fledged, paid members.[20]
One ERC-wide undergraduate program, the NSF-sponsored Research Experiences for Undergraduates (REU) program, initiated in FY 1987, provided a vehicle for involving non-ERC students in the ERC experience during the summer, working alongside ERC researchers and students. Typically, there were 8-10 students in an ERC’s REU contingent. REU students could come from the ERC’s host university(-ies) or another institution, with a strong focus on students from underrepresented minorities. Even in the early years of the ERC Program, several ERCs had an REU program; later on, nearly all did,[21] and they were encouraged through supplemental awards from the ERC Program.
7-B(b) Graduate Education
Graduate education at an ERC differed from then-traditional engineering graduate education in the ways described earlier: participation in cross-disciplinary research teams, focused on an engineered system, in collaboration with industry. Graduate students worked under the supervision of a faculty member associated with the center. To be included in the ERC, all or part of their support had to come from the ERC or from a separately-funded associated project under the strategic plan of the ERC.
The 1995 report, “Highlights of Engineering Research Centers Education Programs,” described a number of graduate education programs developed by the first generation of ERCs.[22] For example, the Center for Net Shape Manufacturing at Ohio State University (Class of 1986) established an industry-supported Fellowship Program to encourage qualified U.S. residents to pursue graduate studies in manufacturing. The fellowship paid $17,000 per year in industry funds directly to the student, with the university covering tuition and fees. The purpose of the program was to ensure that more U.S.-resident engineering students learned teamwork, effective self-expression, and how to communicate with people working at every level of the industrial commercialization process, from manufacturing to sales and customer support.
Similarly, industrial members of the SRC at Maryland sponsored M.S. and Ph.D. graduate students as named Fellows. Sponsors contributed $25,000 to support a Fellow for one year; this amount covered all expenses, including travel. Each Fellow’s academic advisor coordinated project work with a mentor from the industrial research laboratory to ensure close collaboration.
The U.S. Department of Education at that time had an Electronic Materials Fellowship program that was targeted at U.S. citizens. By 1990, nearly a third of the recipients of those fellowships were ERC students.
Although most Gen-1 ERCs had only one host university (with at most one partner university in a few cases), several of these centers awarded fellowships to graduate students at other institutions, including foreign universities in the case of the ERC at North Carolina State University, in an effort at educational outreach.
All the ERCs developed courses focused on their mission areas. By the end of its first year in operation, for example, the SRC had introduced 20 new or modified graduate-level courses emphasizing AI, VLSI, and Computer-aided Engineering. These included a graduate course/seminar on AI tools which was given from their newly developed Applied AI Laboratory.[23]
By the end of its second year, the BPEC at MIT had nine graduate courses in chemical engineering that dealt with biotechnology and biochemical engineering processes. In the first year alone, 164 students were enrolled in these interdisciplinary courses.[24] BPEC’s most prominent accomplishment in education, however, was the early establishment of an Interdepartmental Biotechnology Program, a joint degree program which exposed students grounded in a “home” discipline (Biology, Chemical Engineering, or Chemistry) to rigorous, team-taught coursework by BPEC faculty from the other two disciplines. In the late 1990s and by 2002, this innovation led to significant MIT curriculum reforms in which all MIT undergraduate engineering students are required to take biology, and to new degree programs for undergraduate and graduate students that integrate molecular and cell biology with engineering.
New degree programs began around the end of this early period to be the hallmark of successful ERC education programs. In addition to the curricula at MIT’s BPEC, the SRC at Maryland gained approval in 1990 for a Master’s degree in Systems Engineering.
Although they broke ground that challenged the status quo and, in many cases, posed daunting challenges for university administrators and even some faculty, these graduate programs at ERCs were immediately popular with students. For example, at Columbia University’s Center for Telecommunications Research (CTR), a regular graduate-level course on packet-switched networks registered 85 students in the fall of 1985, a record number for that course. The number of applicants for the Ph.D. program in telecommunications at Columbia “soared to an all-time high” that year, with 200 applicants for research assistantships in the Electrical Engineering Department alone, of which only 12 or 13 could be accommodated.[25]
One of the most effective mechanisms for graduate education in the ERCs has been participation in large-scale systems projects with industry. An early example was Project ACORN (Advanced Communication Organization Research network) at the CTR. This project, jointly funded and conducted by the CTR and ten of its industrial members, drew from research accomplishments in the center’s various thrust areas and integrated them into an experimental communications network. The project served as a testbed for CTR-developed telecommunication network system concepts and also as a focus for new research. Students participating in ACORN experienced a combination of academic research and industrial development in a realistic, hands-on setting.[26]
The Director of the Montana State Center for Biofilm Engineering (CBE), one of the centers in the last cohort of Gen-1 ERCs, in 1996 wrote a white paper on the new characteristics of the contemporary engineering Ph.D. candidate and his/her education, deriving from the innovations being brought to graduate education and research by ERCs.[27]
7-B(c) Role of Industry
Industry was integrally involved in ERC education in many ways from the start of the program. In addition to funding fellowships and testbeds, as described in the previous section, industry representatives often came to campus as visiting faculty, where they taught and mentored students at all levels, as well as participated in research. These visits ranged from a few weeks to as much as three years.
At Columbia’s CTR, more than 20 adjunct faculty from several companies routinely taught CTR-related courses in the departments. They also offered seminars and supervised study for small groups of students. The Center for Compound Semiconductor Microelectronics at the University of Illinois-Champaign (Class of 1986) likewise had an Industrial Visitor residential program that provided the same kinds of services to students while also affording the industrial researchers access to the Center’s state-of-the-art facilities. Industrial Fellows played a similar wide-ranging role at the University of Minnesota’s Center for Interfacial Engineering (CIE) (Class of 1988). Some centers, such as the CIE, formed an Education Subcommittee of their Industrial Advisory Board to help guide the industrial interactions with students and the education program. In later years this feature became more common among ERCs.[28]
The value to ERC students of this kind of exposure to industry practices and practitioners was clear. Every ERC reported that students and faculty alike valued it highly. But there was a reciprocal value for industry in the relationship with ERCs. Through continuing education seminars and classes as well as joint research, industrial participants were exposed to advanced concepts in research and technology. There were many variants on this theme: center seminars and workshops in which ERC faculty presented their research to their counterparts in industry; lecture series featuring distinguished visitors from around the world; and poster sessions in which students presented their research to industry. Perhaps even more importantly for industry members, they were exposed to high-quality young graduates whom they were in many cases eager to employ. It was, in effect, an extended pre-employment audition that in many cases involved internships and summer employment of the students.[29]
7-B(d) Precollege Involvement
During the first five years of the ERC Program, the recognition was dawning that the difficulty of recruiting sufficient numbers of well-prepared domestic students into engineering was a “pipeline” problem originating well before college. But university schools of engineering had little or no experience in addressing this need. In 1988, an NSF-wide program titled Young Scholars was initiated. It was aimed at giving talented secondary-school students an introduction to research in science, engineering, technology, and mathematics and giving them a broad perspective on those fields. Participants would commute to the university daily for several weeks during the summer to participate in hands-on research projects. Several ERCs had Young Scholars programs.
By 1990, no ERCs had yet launched other precollege programs; but the impetus was building, and this area of education began to flourish in 1991, 1992, and beyond, becoming an integral part of the education program of every ERC.
7-C Broadening the Role of Education in ERCs: 1990-2000
7-C(a) Impact of Findings from Evaluations
i. Lessons Learned by Gen-1 ERC Leaders
The first generation of ERC leaders met in a symposium in 1990 to reflect on the progress made in initiating and managing the first generation of ERCs and to derive some lessons learned for the future of their centers and the new ones to come. Education was high on their list of ERC key features because of its importance to their mission to impact the competitiveness of U.S. industry by producing graduates who understood systems design and development, were more familiar with industrial practice, and had experience in taking ideas through the testbed phase of technology development. While they initially thought it would be their research that would have the most impact on industry, Jim Solberg, the Director of the Purdue ERC noted “I think those in the ERCs now recognize that education is probably the principle means of having an impact.” The ERCs were devoting a large amount of energy and resources to “spreading the culture through education.“[30]
Prior to the meeting, Daniel I.C. Wang, the Director of the Biotechnology Process ERC (BPEC) at MIT, carried out a survey of 10 of the Gen-1 ERCs who identified specific needs that must be addressed by engineering education as:
- A critical need for a new type of student—i.e., graduates who can contribute rapidly and innovatively to the competitiveness of the U.S. manufacturing sector
- The need to update industrial personnel—especially R&D practitioners, regarding advances in knowledge and analytical tools
- The need to impact a multidisciplinary, systems-oriented approach—in education as well as in research
- A need to expand the role of industry in engineering education—to improve students’ understanding of engineering systems and practice and industrial needs.
The ERC Directors at the meeting pointed out that engineering education in general did not address those needs and that only ERCs could offer such an atmosphere. [31]
By the fifth year of the ERC Program’s operation, Wang found common themes across the ERCs surveyed that had been developed in response to education challenges posed by the ERC Program:
- Multidisciplinary teaching
- Coupling between teaching and research
- Industrial partnerships in education
- Emphasis on synthesis and design
- Teamwork and team learning
- Exposure to the “real world” of industrial practice
- Lifelong learning capacity.[32]
These ERC Directors and faculty had learned a variety of things:
- An optoelectronics group at the University of Colorado ERC, where students from electrical, chemical, engineering, materials science, and physics were cross-linked and engaging almost daily in research, found that this cross-disciplinary research was an essential part of their educational experience. (Garrett Moddel, Colorado)
- Students at the Brigham Young University (BYU) Advanced Combustion ERC were “really excited about that (multidisciplinary) interaction and participating in very different ways from individual research programs.” (L. Douglas Smoot, BYU)
- Team projects at the Maryland/Harvard Systems Research Center brought design opportunities into the university setting that gave them hands-on experience and feedback from testbeds, such as a six-legged walker by ERC students that took second place in the nationwide Walking Machine Decathlon; or building membrane fingers with tactile sensing to permit robotic manipulation of smooth, nonrectilinear objects. (Roger Brockett, Harvard)
- New courses in cross-disciplinary fields addressed by the ERCs were being added to department course offerings and cross-listed in two or more departments.
- New practice-oriented degree programs, such as the M.S. in Systems Engineering implemented at the University Maryland, were emerging.
- Broader involvement of undergraduates in research during the academic year and summer resulted in faculty realizing how much these students can contribute to research: “These groups are the best undergraduates. They are very bright, very hard working, and tremendously excited about this opportunity. They are able to work full time and they easily work 16 hours a day through the summer. The faculty work hard to meet them every day and keep them challenged”—changing the attitude from: “How in the world am I going to use these people?” to “How many of them can I get??” (Solberg, Purdue)
- Industry involvement in education included bringing industry representatives to the classroom, taking students to manufacturing plants and industry labs, and encouraging industry participation in research and mentoring of students.
- Short courses were being provided for industry personnel on campus to re-educate practicing engineers about the new cross-disciplinary fields the ERCs were developing, but participation was low because industry personnel generally had too little free time to attend.[33]
ii. Effectiveness of ERC Graduates in Industry
NSF’s guidance regarding ERC education programs in the second decade of the ERC Program was informed by a contract to Abt Associates, Inc. in 1994, which was designed to assess broader impacts of an ERC experience on its graduates and their employers as they moved into the workplace. The Abt team surveyed ERC graduates (M.S. and Ph.D.) from the first generation of ERCs who were working in industry, academia, and Federal laboratories. The sample pool included students from all 18 ERCs that had been funded between 1985 and 1990. They also surveyed their supervisors. A total of 720 survey instruments were mailed to graduate alumni/ae of the ERCs, with a 60 percent (433) response rate. The responses were classified by sector—industry 61%, academic 56%, and other (generally Federal Labs) 64%. These graduates voluntarily identified 554 supervisors, of whom a total of 477 (86%) completed interviews. The findings “shed light on overall patterns and hint at certain cause-and-effect relationships.”[34]
The results of the surveys of the ERC graduates are consistent with the hypothesis that ERC graduates demonstrate superior performance on the job compared to peers who were not affiliated with ERCs—i.e., high scores on self-ratings and supervisors’ rating of job performance compared to peers across all sectors—but it cannot be ruled out that this may be due to their overall higher quality in general.[35] In addition, insights from the graduates about the impact of an ERC experience showed that:
- 82 percent of graduates rated their ability to apply knowledge from different disciplines as better than that of their peers and more than half said that their ERC experience had a positive impact on this performance.[36]
- 60 percent of doctoral graduates rated themselves “as much better than average” in their contributions to their company’s technical work, compared to 40 percent of ERC graduates who had not had this prototyping [testbed]experience.[37]
- 44 percent said they experienced a high or extremely high involvement in a systems approach to solving engineering problems as students, while 44 percent said there was a moderate involvement – for an overall 88 percent systems impact.[38]
Surveys of the supervisors of ERC graduates produced evidence that the ERC experience did result in new employees who were more effective in industry and government laboratories. In addition, overall there was an improvement over time between the ERCs surveyed in 1994 (Gen-1) and those surveyed in 2001(some of Gen-2). Each of the features in Table 7-1 represents features of ERCs that are important to industry. In the first cohort (1994), ERC-trained employees performed from 53 to 80 percent better than single-investigator trained employees and from 64 to 87 percent better in the second cohort (2001). The most significant increase in performance of 24 percent between the first and second cohorts was the ability to work in interdisciplinary teams; secondly, ERC graduates in the second cohort required 22 percent less training to become effective contributors to the firm; and thirdly, the ability to apply an engineered systems perspective rose by 17 percent between the two cohorts. The ability to work in interdisciplinary teams and have a systems perspective are the two most significant key features that distinguish ERC-trained graduates from single-investigator-trained graduates. Clearly, an ERC experience had significant payback for the firms who hired these graduates.
Table 7-1: Comparison of Performance of ERC Graduates with Comparable non-ERC Hires (1994/5 and 2001/2) [39]
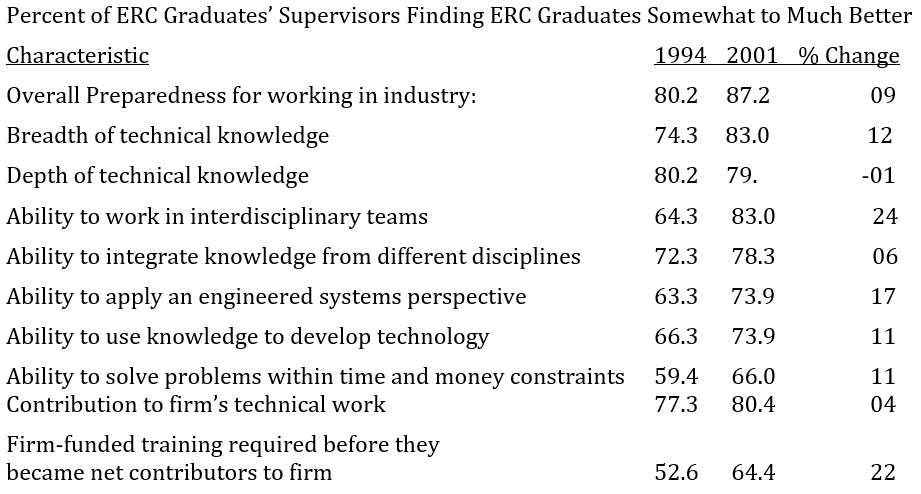
7-C(b) Increased Emphasis on Education and Diversity
i. New Leadership of the Directorate for Engineering
The results in Table 7-1 corroborated the lessons learned by the Directors of the first cohort of ERCs and validated the goals set out for ERCs by the Program guidelines. However, there was more work to be done in ERC education and its broader impact on engineering education nationwide. Figure 7-1 displays the changes in ERC education program that took place between 1990 and 2000.
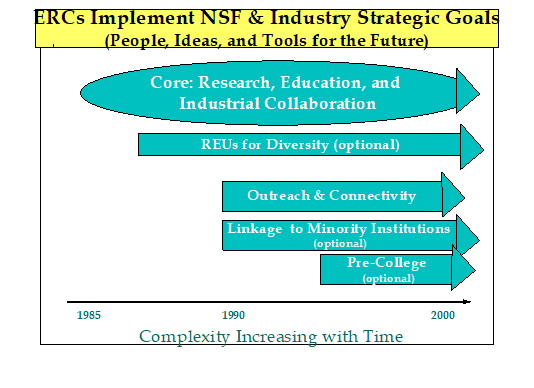
Figure 7-1: Evolution of ERC Features 1985-2000
With the arrival in 1991 of a new Assistant Director for Engineering, Joseph Bordogna, who had a strong personal and professional interest in engineering education, the ERC Program was complimented on its achievements in education and outreach but challenged to expand the educational impacts of ERCs to (1) a wider set of universities and (2) more diverse cadres of engineering students. This broader impact on engineering education had to be achieved within the constraints of the existing ERC budget, which meant that large numbers of new ERCs could not be supported. To achieve that impact, ongoing and new ERCs were required to have outreach partnerships with colleges and universities, including some that served populations predominantly underrepresented in engineering. These diversity-focused outreach efforts were not mentioned in ERC program solicitations until the FY 1993 solicitation, resulting in the Class of 1994-95.
ii. ERC Outreach and Connectivity Beyond Partner Institutions
In addition to involving on-campus undergraduate students in research, ERCs also reached out to undergraduate students across the country to involve them in the excitement of research in an interdisciplinary team culture. With the start of the NSF-wide Research Experiences for Undergraduates (REU) program in 1997, ERCs were encouraged to submit proposals to that program and were offered supplemental support to establish summer REU programs through the ERC Program as well, since there was concern that there might be a negative bias against center vis-a-vis single-investigator awards, given the size of an ERC’s budget and its stipulated education features. These efforts stimulated interest in the ERC’s field of research among a wider spectrum of students, often including students from population groups traditionally underrepresented in engineering, such as women, African Americans, Hispanic Americans, Native Americans, and persons with disabilities.
By the 1997 ERC solicitation, this outreach was broadened to enable an ERC to develop a flexible program of connectivity and outreach that could include foreign universities and collaborations with universities serving predominantly underrepresented groups in engineering or technical colleges.[40]
Outreach to involve other schools in the region or the Nation in the ERC as appropriate, such as summer research program for undergraduates from other universities, and joint research projects with other universities where complementary expertise or facilities are available. Generally, these were summer outreach efforts until the introduction of the WWW, which enabled year-round remote collaboration in joint research projects across campuses.
The ongoing ERCs were challenged to increase the diversity of their students through more expanded inclusion of on-campus students and outreach. Because of the overall lack of diversity in the Duke engineering school, the ERC at Duke was challenged to develop outreach programs to bring students from underrepresented groups to participate in its cardiovascular biomedical research programs. To address this challenge, the ERC hired Martha Absher to manage effort. She had been educated in mathematics at Berea College in Kentucky, a college devoted to bringing higher education opportunities to racially- and gender-diverse students from Appalachia. Initially the Director of the ERC asked Absher to focus on the disabled. In response, she developed an REU outreach partnership with Gallaudet College in Washington, DC, which is devoted to higher education for the hearing impaired. The Gallaudet students came on the Duke campus with signing assistants to facilitate communication. Given the success of that effort and the continued push from NSF to make the ERC more racially diverse, Absher was asked to manage both outreach efforts. She expanded the outreach effort to include universities serving predominantly African-American students from North Carolina A&T University, North Carolina Central University, and Prairie View A&M University in Texas. Duke’s College of Engineering describes Absher’s efforts as follows:
She was devoted to working with faculty so they became committed and effective mentors and she “broke” the traditional mold of an REU program and asked the same students to come back to the ERC for one or more years to fully develop their skills and confidence. Martha’s philosophy of inclusions is below:
“I want our programs to be inclusive of everybody. This includes underrepresented minorities, defined by the National Science Foundation as African American, Native American, Hispanic, Pacific Islander and Native Alaskan. I’ve had students from all these groups represented in my program over the years. People with disabilities often aren’t included in diversity programs. I wanted to make a special commitment to get them involved. Students with disabilities have made up 25 percent of our NSF REU students.”
Since the program began in 1989, Duke Engineering has hosted 341 undergraduates as part of the REU program. Many of those students have gone on to distinguished academic careers, and they credit the REU program with putting them on the right track.
Tyrone Porter, an REU student in 1994 from Prairie View A&M University in Texas, studied biomedical engineering with Patrick Wolf, associate professor of Biomedical Engineering, that summer.
“Dr. Wolf exposed me to the diversity of BME research, and I was hooked,” Porter said. Porter went on to get a PhD in bioengineering at the University of Washington and is now an associate professor at Boston University. He visited Duke in 2013 as a faculty guest lecturer to present a BME seminar on ultrasound.
For Daniel Lundberg, a deaf student from Gallaudet University who participated in the REU program in 2001 with Fan Yuan, professor of Biomedical Engineering, the experience helped him with research skills. But, more than that, he learned how to better use American Sign Language to work with interpreters and communicate with hearing audiences, which was essential to his future professional development.
“It helped me connect with the audience better. After that experience, I was a changed man when it came to presentations—one of the best rather than one of the worst,” he wrote in an email. Lundberg is now an associate professor of chemistry at Gallaudet University, a higher education institution for deaf and hard of hearing students. [41]
Another unusual ERC outreach program, the Multimedia University Academy (MUA), sponsored by the Integrated Media Systems Center (IMSC) (Class of 1996) at the University of Southern California, opened its doors to its first students on September 3, 1997. The IMSC MUA was established using leveraged funding from a number of units within NSF, as well as from the Shaye Foundation.
This education program was designed to meet several objectives:
- To meet inner-city industry demand for a workforce trained in multimedia and creative technologies and to provide a transition program to careers in multimedia production technology for out-of-school youth at risk for poverty because of deficient academic skills and a lack of career goals that prepares them for advanced education and employment; and also
- To link the basic engineering systems research undertaken by the IMSC to effective instructional application by providing an appropriate testbed for intelligent tutorial systems and establishing a program to study multimedia-based instructional modules and methods.
iii. Expanding Pre-college Education in ERCs
As the leader of a program whose mission centered on strengthening U.S. competitiveness, Lynn Preston was concerned about where the next generation of engineering undergraduate and graduate students would come from; there was an increasing tendency to admit foreign graduate students to U.S. engineering schools, who were under no obligation to stay here once they graduated. She was concerned that middle and high school students who were more talented in science and spatial, analytical, and mathematical reasoning often found little outlet for those skills in an educational system that was becoming increasingly skewed toward verbal skills, at the expense of what we call today STEM (Science, Technology, Engineering, and Mathematics). She firmly believed that there were (and are) students in middle and high schools who are “destined” to be engineers but don’t know it. They don’t know it because in those days, prior to the emphasis on STEM education encouraged by NSF, they would have little or no experience with engineering concepts at home, in the classroom, or in other environments where they spend time—whereas prior generations learned engineering by building radios, fixing machinery on the farm, building go-carts, repairing cars, etc.
Preston was also inspired by the ERC for Intelligent Manufacturing Systems at Purdue, which had developed an outreach program with a West Lafayette, Indiana, middle school teacher. Purdue donated a CNC machine to the school and students learned to develop computer code to control the machining of small keychains and the like. Faculty and students from the ERC went to the school to work with the students and serve as role models. As the Purdue ERC and outreach team explained in their presentation to one of the ERC Program annual meetings, these students became intrigued by the design and “manufacture” process and thus became more interested in their mathematics classes. This effort pointed to new ways of engaging high school and some middle school students and some of their teachers in activities designed to expose the students to the design process and “making” things.
Slowly the ERCs began to broaden their involvement with pre-college students and these ERC pre-college education programs engaged ERC faculty and students as mentors of the pre-college teachers and students, resulting in long-term relationships between ERCs and nearby schools. Another example was the Caltech Classroom Connection, developed by Eddie Branchaud, an ERC graduate student at the Caltech Center for Neuromorphic Systems Engineering (Class of 1995), who, on his own, organized Caltech students to go into the Pasadena Public School District to teach in the classrooms. Before this, Caltech had not engaged the local school systems in outreach efforts in science and engineering. (See Section 7-E(a).)
At the ERC at Northeastern University, the Center Director, Michael Silevitch, had been actively engaged in bringing engineering concepts to the pre-college classrooms before he was awarded the Center for Subsurface Sensing and Imaging Systems in 2000. From the Education Programs chapter of the ERC Best Practices Manual:
The Center for Subsurface Sensing and Imaging Systems (http://www.censsis.neu.edu) has an ambitious education program, to which the director is passionately devoted. The center’s K–12 Education Outreach program has been designed, developed, and implemented in partnership with the Center for the Enhancement of Science and Mathematics Education (CESAME) at Northeastern University http://www.cesame.neu.edu.[42] After 12 years of experience in education programs, the director understood the importance of partnering with other organizations to share their expertise and use their network of contacts. Doing so saves time and resources and allows the programs to take advantage of the credibility of these established entities.
In selecting projects for their program many aspects are considered, so that a project is not an end in itself. One goal is to have a lasting impact—addressing a real problem rather than just creating a momentary interlude. A second goal is to improve the number and diversity of students moving along the engineering pathway. Finally, the project should connect to the ongoing work of the center.
One project that has taken place in the past two years (2000–2002) is the CenSSIS Challenge “Hidden Worlds” for high school students and their teachers at the annual Massachusetts Pre-Engineering Program (MassPEP) competition. Project coordinators develop a different challenge each year. In 2002, student teams used remote digital cameras to capture information and, from a photo-mosaic, identify objects and structures and measure distances between objects. Prior to the competition, the teachers and students were provided with a digital camera and printer and a list of suggested activities to become familiar with the specifications of the camera and practice the skills necessary to complete the challenge.
This project meets the education program’s goals by providing equipment and technology, thus addressing the real problem of infusing technology into the schools. By participating in the MassPEP event, with its extensive network of involved urban schools, diverse student groups are introduced to the engineering pathway in a fun and exciting way. The content of each challenge is based on real problems faced by the center’s partner institutions, in this case the mapping of coral reefs.
In the future the center hopes to distribute the project through their partner institutions to other urban areas. Currently the challenge project is funded through a grant from the Massachusetts Department of Education. The center will use core funding if necessary to continue the project, however, because of its systemic impact.[43]
Some ERC students became actively engaged in designing new materials for pre-college outreach and in direct interaction with the pre-college students. The University of Washington Biomaterials ERC (UWEB) developed what was, for its time, an innovative interactive bioengineering game called “Guy Simplant,” that provided an educational component as students helped the cartoon figure Guy rebuild his injured body parts, and made CDs copies available for wide distribution.[44] An online version is still available as of this writing that can be used to teach heart health and rebuilding of injured body parts, such as a missing hand. (See http://depts.washington.edu/simplant/ )
iv. ERC Engineering Efforts in the Context of NSF Engineering Education in the 1990s
During the 1990s, the Directorate for Engineering became more fully engaged in engineering education, broadening the impact of what could be achieved through ERCs. The Engineering Education Coalitions were established in 1990. Their goal was to mimic the broad, revolutionary goals of an ERC but focus them on engineering education, not through a center but through a coalition of university efforts funded under one umbrella award. In addition, in 1992, the home division of the ERC Program and the Industry/University Cooperative Research Centers Program (then called the Engineering Centers Division) was expanded to include engineering education more broadly. The division was called the Division of Engineering Education and Centers (EEC). This moved the budget for the Coalitions and their staff into EEC. Sue Kemnitzer led the Education Programs of EEC and Lynn Preston led the EEC Center Programs as Deputy Division Directors. By the 2000s, funding for the Coalitions wound down and the funds were reprogrammed to initiate support for innovations in engineering education at the non-center and center levels, including the Centers for Learning and Teaching, which were headquartered at the University of Washington and Utah State University.[45] These efforts were closely coordinated with the staff of the engineering education programs in the NSF Directorate for Education and Human Resources (EHR).
v. Broader ERC Impacts on the Engineering Curricula
Since only a small percentage of all U.S. engineering students can directly participate in an ERC, these centers are required to infuse engineering curricula with their interdisciplinary, systems perspective and to disseminate these curricular innovations throughout academic engineering at the undergraduate and graduate levels. Between 1985 and 2016, ERCs produced 120 new degree and certificate programs, 986 new courses, 3,118 modules and course materials to impact ongoing courses, and 170 textbooks. These educational materials and degree programs impacted all the core fields of engineering and many have supported subfields, such as power electronics and electronic packaging, and helped establish emerging fields such as biological engineering and multimedia engineering.
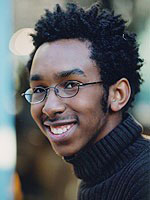
André Green was a senior in the first class of MIT Biological Engineering majors, graduating in 2000, and received his Ph.D. in 2014. |
An example of the broad educational impact of ERCs is the role of ERCs in the development of the emerging field of bioengineering. NSF funded 15 bioengineering ERCs between 1985 and 2017. Together these ERCs have provided a new interdisciplinary paradigm facilitating the creation of the field of bioengineering as well as an educational foundation for bioprocess engineering, tissue engineering, biomaterials engineering, biomimetics, and synthetic biology, among others. Intensive training in both biology and engineering is required to enable bioengineering graduates to work at the cusp of these fields to advance knowledge and technology for the delivery of advanced diagnostics, medical treatments, pharmaceuticals, and biomimetic prostheses.
ERCs can also have broad and powerful impacts within an institution. An example is the MIT Biotechnology Process Engineering Center. Not only has BPEC had a long-lasting and broad-based impact on the field of bioengineering, but it has also strongly impacted the culture of MIT and MIT’s graduate and undergraduate education programs. This impact took nearly 20 years to be fully realized. BPEC, established in 1985 and led by Professor Daniel I.C. Wang, was the first ERC to focus on the interface of biology and engineering in order to advance education and technology for the biotechnology industry. (It is the only ERC to successfully compete for and complete a second 10-year term of support.) BPEC initiated research teams that involved engineers, biologists, and chemists—something out of the ordinary at MIT (and elsewhere) at that time—and this fostered team teaching across these disciplines, leading to a new interdisciplinary education and research culture at MIT. To support this culture, in 1998 MIT established “Biological Engineering,” the first formal academic unit linking biological science and engineering departments, led by Professor Douglas Lauffenberger, the second BPEC Director. This led to a new Bioengineering Ph.D. program, established in 1999. Professor Linda Griffith joined BPEC in 1998 and began a campaign to strengthen the educational underpinnings for the field of biological engineering at MIT. In the late 1990s she became the third Director of BPEC and led an interdisciplinary team of professors who convinced MIT to establish the first new undergraduate major in 39 years at MIT, the Biological Engineering “Course 20.” About 125 students were enrolled in 2002 and the first class of 23 graduated in 2008.
The Center for Computer-Integrated Surgical Systems and Technology (CISST), headquartered at The Johns Hopkins University, prepares engineering students to invent and develop advanced medical technologies. CISST’s “Surgery for Engineers” course, established in 2002, engaged students in new and exciting learning experiences, built bridges between engineers and medical practitioners, identified and solved real-world problems using engineering principles, and strengthened the undergraduate curriculum in terms of career preparation. This course taught engineering students the fundamental skills and operative procedures for general surgery. It was a hands-on course designed for engineers who are developing computer-integrated surgery tools that will improve upon current technologies in use in the Operating Room.
Undergraduates at the University of California at Berkeley expanded a competition they started at thePacific Earthquake Engineering Research Center (PEER) to involve their peers at other institutions, as well as professionals in the field. PEER’s Student Leadership Council organized the annual Undergraduate Shake Table Competition in 2004, challenging civil engineering students to construct structural models that perform well under earthquake simulations. Practitioners and judges rated teams on an oral presentation, the design’s performance, technical merit, and economics. In 2005, the PEER students invited into the competition their peers from the MCEER at the University at Buffalo and from the Mid-America Earthquake Center at the University of Illinois at Urbana-Champaign, two other ERCs. In 2006, the students took the competition to the national level—at the 8th U.S. National Conference on Earthquake Engineering, where it attracted an audience of professionals and conference sponsors. This competition gave students opportunities to test out and gain a better understanding of what they had learned in the classroom. Competing at a national conference exposed them to career opportunities in earthquake engineering and gave them a chance to mingle with professionals who could prove to be good contacts later on.
The Integrated Media Systems Center (IMSC), an ERC established in 1986 at the University of Southern California (USC) and now graduated from ERC Program support, has had a profound impact on USC’s education programs. The Center works at the interface of engineering, computer science, and the media arts. As a result, the ERC created and maintains nine academic programs. They are:
(1) BSEE in Integrated Media Systems—Started in Fall 2002; emphasizes creative technical aspects of multimedia;
(2) Minor in Multimedia and Creative Technologies (MCT) (for engineering students)—Started in Fall 1996; emphasizes technology but with breadth across computer science, electrical engineering, cinema-television, music, and fine arts;
(3) Minor in Interactive Multimedia (for non-engineering students)—Started in Fall 1997; includes a computer literacy component, as well as breadth across computer science, electrical engineering, cinema-television, journalism, and fine arts, but with less emphasis on deep technology issues than the engineering-oriented minor;
(4) Minor in Video Game Programming (engineering students)—Started in Fall 2004; integrates theoretical concepts and practical skills to prepare students for a career in interactive entertainment (i.e., the video game industry through integration of Computer Science and Information Technology;
(5) Minor in Video Game Design and Management (non-engineering students)—Started in Fall 2004; integrates theoretical concepts and practical skills to prepare students for a career in the video game industry;
(6) High School Summer Program in Video Game Production (engineering and non-engineering students)–Started in Summer 2004; provides high school students the opportunity to work in the IMSC Serious Games Lab assisting researchers and lab assistants on games research projects;
(7) Master of Science in Electrical Engineering with a Specialization in MCT—Started in Fall 1996 and substantially modified in Spring 2000; differs from general MSEE in that students are required to take four multimedia technology courses and must focus on digital media processing or interactive networks;
(8) Master of Science in Computer Science with a Specialization in MCT—Started in Fall 1996; differs from the general MS CSCI in that students are required to take one multimedia technology course, after which they must focus on graphics and vision, multimedia information management or networks;
(9) Master of Science in Integrated Media Systems—Started in Fall 1998; the program breaks down barriers between CSCI and EE and students take formal training in multimedia application tools, so that they can create a portfolio in four sub-fields of digital media processing and hardware design (EE), graphics and visualization (CS), multimedia information management (CS) and interactive networks (EE/CS). Students get breadth and depth across at least two of the four areas; directed research is encouraged.
vi. ERC Program Funded Experiments in Engineering Education
1. Education for Innovation
From the start of the ERC Program, center researchers and students occasionally spun companies off from the centers. To examine the possibility of educating students to be more successful in this regard, in 1995 the ERC Program funded a pilot project at NC State, the Technology Education and Commercialization (TEC) Program, that was intended to teach the necessary skills for technology entrepreneurship. Professor Angus Kingon, from the NC State business school, who was affiliated with the NC State ERC for Advanced Electronic Materials Processing, was the PI. Classes included both students and faculty from the University’s engineering and management schools, which were jointly offered by both schools. Soon after students began enrolling in TEC courses, existing firms began enrolling their employees so that the latter would learn to be entrepreneurial within their firms—entrepreneurs who could identify and manage successfully technologies with strong revenue potential. In the three years after the completion of the ERC funding, the TEC Program PIs responded to firms’ increased awareness that their competitiveness is strongly linked with systematic management and exploitation of intellectual property by adapting their basic approach to systematic assessment of intellectual property, embodied in the TEC Algorithm, to include a portfolio management module. The PIs also introduced TEC Clinics, in which they teach corporate technology managers how to value their intellectual property by finding product manifestations that accounting and management can recognize as an asset and how to use the Algorithm for portfolio management.[46]
2. VaNTH
During this period (1990-2000), the ERC Program supported an experiment to see if the ERC model could be used to effectively focus a team on the development of new curricula and learning technologies to support an emerging field, in this case biotechnology. As an outcome of a solicitation,[47] the VaNTH (Vanderbilt-Northwestern-Texas-Harvard/MIT) ERC was funded, under the leadership of Professor Thomas R. Harris, an MD/Ph.D. from Vanderbilt. The “driving forces” behind this decision are shown in Figure 7-2.[48]
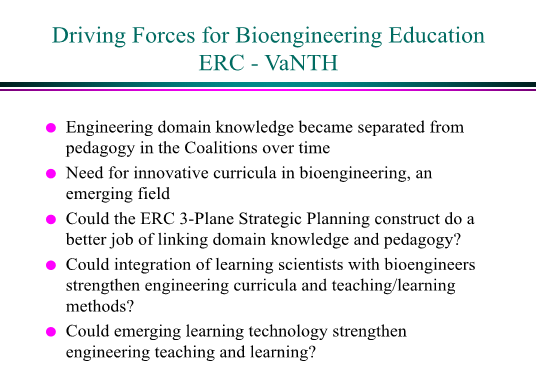
Figure 7-2: Why a Bioengineering Education ERC?
The goal of VaNTH was to produce adaptive experts in bioengineering, educated through a challenge-based framework. The ERC brought learning science and learning technology principles to bioengineering education for the first time at the center level. VaNTH developed the Courseware Authoring and Packaging Environment (CAPE), a learning technology for integrating learning science and bioengineering to facilitate the development of courses using the VaNTH learning frameworks (Figure 7-3).[49], [50] During its time in the ERC Program, VaNTH produced 21 new courses, 250 modifications of ongoing courses, 2 texts, 241 course modules, 1 new degree program, 6 minors, and 6 certificate programs. One of the major innovations has been approximately 50 modules and courses (containing multiple modules), posted on the VaNTH Portal, which were available for faculty outside the ERC. These covered deliverables available beyond VaNTH in many of the major areas of bioengineering, including biomechanics, physiology, optics, and biotechnology—which were the first domains to receive attention—as well as signal analysis, transport, imaging, and core competencies such as writing. Materials to teach full courses were available in biomechanics and transport. A reference to VaNTH CAPE tools is posted on the web at http://w3.isis.vanderbilt.edu/Projects/VaNTH/Index.htm.
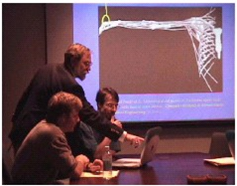
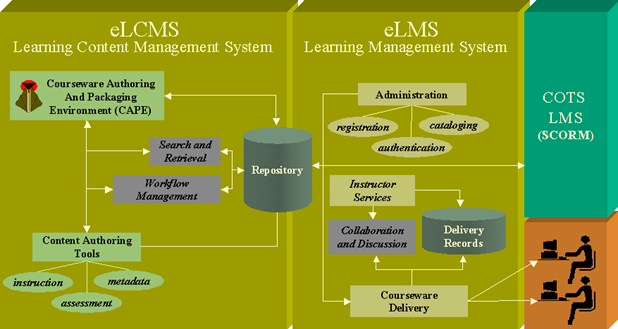
Figure 7-3: VaNTH Learning Content Management System[51]
Sean Brophy was a learning sciences research faculty at VaNTH. He is now an Associate Professor of Engineering Education at Purdue University and notes: “I worked on a couple of projects to use the CAPE/eLMS system to support blended learning in the First Year Engineering experience at Purdue. I’ve also been working to expand the use of our Challenge Based Instructional approach using HPL and STAR Legacy frameworks in various other domains including civil engineering, electrical engineering and mechanical engineering. I’m now working with these ideas to formulate a physics-based intro to engineering course for first-year honors engineering. VaNTH was an outstanding experience and I enjoyed working with everyone to advance bioengineering/biomedical engineering education.”[52]
As VanNTH graduated from NSF support, its Director, Tom Harris contributed these insights and lessons learned:
- Bioengineering was a subject ripe for further examination, as it is a complex subject requiring synthesis of biology, medicine, and engineering; the investment in VaNTH to study what should be taught and how to teach it was timely and important for the growth of the field.
- Developing an organizational structure that would attract research faculty to explore methods to improve their teaching was an important feature of VaNTH and the prestige of an ERC helped to attract strong faculty—academic leaders who could raise the priority of education research and improvement to a high level.
- Joining engineering researchers with learning scientists, assessment experts, and learning technologists worked well once significant terminological and other barriers had been surmounted.
- The learning science community (on the VaNTH team and on the NSF site visit team) brought a fresh awareness of issues in engineering education.
- The ERC notion of “testbeds” was quite applicable to education research, especially across several institutions. We were able to produce good experimental design that actually measured student achievement in living context of actual classes, thus avoiding the anecdotal nature of much education research.
- The incorporation of the How People Learn Framework helped focus our learning technology group.
- The ERC industrial partner system did not function well for an education ERC, essentially because industry doesn’t see education as its primary mission.
- The ERC review process is extremely burdensome.
- For future progress, domain expertise in bioengineering should not be divorced from learning theory and pedagogical innovations.[53]
7-C(c) Strengthening ERC Education Programs
i. Strengthening Education Program Leadership
During this period, the ERCs began to appoint education program directors from staff or faculty, as the scope of the ERC education programs expanded and NSF’s expectations became more stringent regarding education.[54] Education program directors became a required component of an ERC’s leadership team in FY 1994.[55]
During the 1990s, a community of ERC education program directors emerged who shared their challenges, successes, and failures at successive ERC Program Annual Meetings. A key leader of this community emerged—Anne Donnelly, who served as the Associate Director for Education and Outreach at the Particle Engineering Research Center at the University of Florida, Gainesville. She has a Ph.D. in science education so she understood both pedagogy and science. She and the ERC’s Center Director, Brij Moudgil, formed an effective partnership integrating research and education at all levels of the ERC, including educating practitioners. Other Education program leaders emerged to serve a role in leading this community. One was Beth Tranter, at the Virginia Tech ERC for Power Electronic Systems, who worked tirelessly to build partnerships with underrepresented minority-serving institutions within this ERC. Another was Fanaye Turner, the Education Director at the University of Washington’s UWEB ERC, who was especially talented in recruiting precollege teachers and their students to the ERC, where the students were given research projects to pursue in their classrooms. The team of ERC education leaders began to form some best practices based on their shared experiences, which they decided to publish through the first ERC Best Practices Manual. The Education Programs chapter was written in 1998-1999 and published together with chapters on the other ERC key features.[56]
The Education Directors recommended the following regarding the role of the education program director:
The choice of education coordinator/director, and the appropriate positioning of this person as a member of the center’s leadership team, will determine the success of the center’s education program. In some centers, the management believes that this person should have a PhD, to elicit the full respect and cooperation of faculty. This is especially important for curriculum development. This question should be considered and resolved at the outset by the center director and the executive committee. In any case, the primary focus should be on identifying an individual with an appropriate background to be responsible for the education activities of the center. His or her interest in interacting with students should also be a major selection factor.
Education coordinators/directors are responsible for writing up all aspects of their education programs for the ERC annual report and other documents. They also develop and write grant proposals of many types to expand their education programs. Therefore, strong communications skills and an ability to prepare successful proposals are important.
It is recommended that an education advisory committee be established to give center faculty a mechanism to provide input into center education programs and to provide support for them. The composition of this group can include center faculty, external faculty, and industrial partners as is deemed appropriate. [57]
ii. How to Start Up an ERC Education Program
Among the issues to be considered in developing and implementing the education plan for an ERC, the Education Program Directors recommended:
- development of an education vision and goals (both short-term and long-term) consistent with the center’s strategic plan and objectives;
- identification of the particular populations and student groups to be impacted
- assessment of resources, both personnel and financial;
- development of guidelines for undergraduate and graduate student participation
- development of a recruitment strategy for undergraduate and graduate students, recognizing that different strategies are required;
- design of an outreach strategy to include K–12, undergraduate, graduate, industry, community, etc., short-term and long-term, which meets NSF and center goals;
- development of a plan to recruit and retain underrepresented populations in engineering (women, underrepresented minorities, persons with disabilities) in the center’s education and research areas;
- development of a strategy to infuse ERC research findings into the curriculum;
- development of mechanisms to integrate students into all aspects of the center;
- development of mechanisms for industrial interaction at all student levels, graduate and undergraduate; and
- development of a schedule of ERC courses, seminars, and other academic requirements so students can commit to them early in their careers.[58]
iii. NSF’s Support for ERC Education Programs
Education programs were financially supported through the base ERC budget. In addition, an ERC could apply to the ERC Program for a supplement to augment its funds devoted to an REU program. NSF’s Mary Poats managed that supplemental support throughout the 1990s and Esther Bolding took over that role in the early 2000s as Mary began to focus on developing and managing the Research Experiences for Teachers Program. Esther was also responsible for the ENG-wide REU program that funded sites and supplements, under the NSF REU Program, until her retirement in 2014.
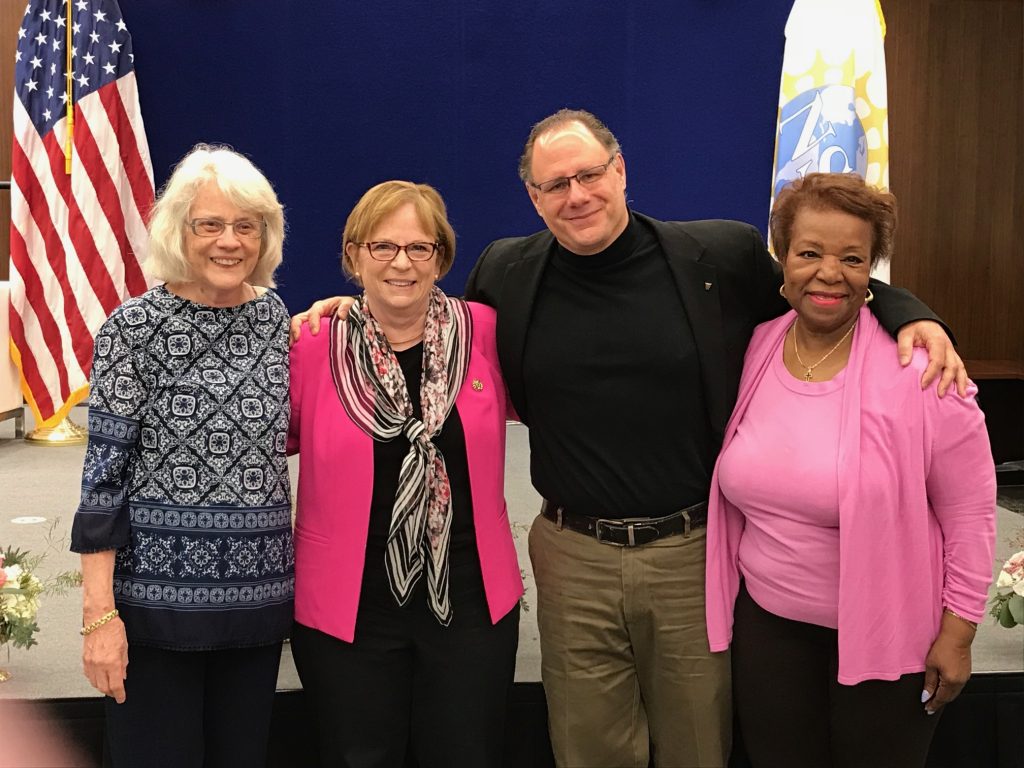
Mary Poats, second from left, upon receiving the NSF Distinguished Service Award in May 2018. From left are Lynn Preston, Poats, Don Millard (EEC Division Deputy Director), and Esther Bolding.
The ERC education program leaders noted that:
Personnel of the NSF Engineering Education and Centers Division are very obliging in helping the ERC education coordinators/directors develop and enhance their education programs. They have the experience to provide guidance and identify others who might serve as resources to assist in strengthening the education programs. NSF also provides publicity to industry and works through other NSF programs to support the centers.
…one of the key ways that NSF has helped, and will continue to help, is in the area of supplemental funding based on competitive proposals. The funding provided serves as a nucleus for developing strong education programs. More recognition of the importance of ERC education programs in the ERC annual meetings and during site reviews will help education coordinators/directors to strengthen their respective education programs. NSF support is philosophical as well as financial and is critical for developing strong ERC education programs and ensuring that education is an important aspect of the centers.
Some examples of NSF’s aid to ERC education programs are these:
- By continually stressing the importance of education and educational programs in the ERC, NSF program directors and officials emphasize to the leadership and faculty of the ERC the significance of these programs. This greatly helps the education endeavors at the centers.
- NSF provides opportunities for additional sources of funding and publicizes these funding options to the centers.
- NSF provides the supporting framework for coordination between centers.
- NSF realizes the significance of collaboration between centers and encourages these collaborations verbally and through funding sources.
- NSF provides critical insight to our centers through the annual site visits that help improve center programming.
- NSF provides guidelines that define the programs from the inception of the center to the reporting guidelines that document annual progress.
- By mandating an industrial component to the center’s architecture, NSF has laid the groundwork for the development of education programs with a strong industrial element, benefiting undergraduate and graduate students.
- By funding the ERC centers, NSF is promoting innovative programs that allow cutting-edge technology to be developed to the point where it can be utilized by industry and benefit the general population. Center education programs are an essential vehicle for disseminating these new technologies into industry, by means of the center graduates and outreach.[59]
iv. ERC Consultancy
To ensure that new ERC Education Directors, either from a new ERCs or those who were new to ongoing ERCs, had sufficient guidance to be successful beyond what they could glean from the ERC Best Practices and the ERC Program Annual Meetings, Preston and Poats initiated the ERC education consultancy. Experienced Education Directors were supported to form teams of other ERC education program leaders to visit ERCs with new education program leaders to assess their current programs and provide guidance to them and their Center Directors on how to start-up or strengthen their programs. In this way, the consultancy members’ expertise could supplement the expertise of site visit review teams to ensure timely advice to help ensure continually strong education programs.
7-C(d) Increased Leadership Experiences for Students
i. Initiating Student Leadership Councils (SLC)
Preston initiated the Student Leadership Councils (SLCs) in 1996 as an outcome of attending numerous site visits, where she noticed that the ERC students were not assuming leadership roles as key members of ERC teams—certainly not a traditional role for engineering students, but one that was expected in an ERC. Opportunities were lost to impact the strategic planning of research and education for the center as a whole and interactions with industry and to build the ERC student body as a community and a component of the ERC organizational unit. Consequently, each ERC was required to set up an SLC and appoint officers. The SLCs were required to meet to provide input to the faculty leaders and industrial members about how to improve their ERC experiences. As a part of this process, they were required to prepare a SWOT (Strengths, Weaknesses, Opportunities, and Threats) analysis and present it to the annual or renewal site visit teams. Through this process, students learned how think as members of an organization and gained leadership experience and knowledge about how to carry out surveys and analyze and present results—skills that would prove valuable in the short run and in their future careers. The SLCs became the social hubs of ERCs as well, as they organized student retreats, pizza parties, etc. Later in time, the ERCs were required to support their SLCs with budgets to support these activities, etc.
Love Blooms at SLC Meeting In 2010 Portia Taylor, then a PhD candidate at the Quality of Life Technologies (QoLT) ERC at Carnegie Mellon University, was the President of QoLT’s Student Leadership Council. Satish Singh was a PhD candidate at the University of Pittsburgh and President of the SLC at the ERC for Revolutionizing Metallic Biomaterials (RMB). Both attended the Student Retreat held in conjunction with the ERC Program’s Annual Meeting in Bethesda, Maryland, that year. They met at breakfast and, in the sharing of mutual professional interests, also found a mutual personal interest. As Portia later said, “The rest was destiny!” It helped that both students’ universities were located in Pittsburgh. Fast-forward to November 2014 and the two became engaged, then married in May 2015. Portia went to work for Philips Research in New York City, while Satish took a research position at Avon in New York. Later he moved over to Philips as a Research Biomaterials Engineer, and Portia became a Senior Research Scientist there. In summer 2018, the couple announced that they were expecting their first child. Although there have been many ERC-generated romances across the years, this is believed to be the first involving a couple from different ERCs, and certainly the first between two SLC presidents. The ERC Program is proud of the thousands of high-quality graduates that it has produced, but it is especially happy to have provided the venue for this auspicious meeting! Drs. Satish Singh and Portia Taylor Singh |
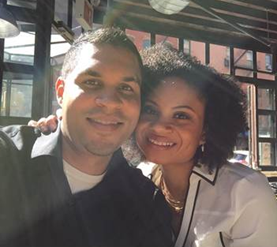
7-C(e) Strengthening Industrial Involvement in Education
i. Strong Interaction of Industry Sponsors with Students
One of the reasons for the findings that ERC graduates are better prepared for success in industry is the fact that they have significant contact with industry personnel while still students. This is one of the attractors for membership in ERCs since it gives industry members a “window” into the competence and readiness for industrial practice of future potential employees. The ERC education leaders summarized ways they have set up for direct interaction between students and industry personnel:
Industry is involved in all aspects of the ERC education program. Industry representatives may serve as mentors to undergraduate, outreach, or graduate students. They may present lectures, course sections, or entire courses, or teach courses in partnership with ERC faculty members. Industry experts may serve on the student’s masters or doctoral committee. Industry may sponsor undergraduate or graduate internships in industry, or sponsor students’ undergraduate or graduate degrees in whole or in part. Industry input will help shape curriculum, develop original courses, and shape the very nature and approach of the engineering curriculum of the future. Industrial representatives may serve on review panels evaluating and shaping the ERC education program. Industry interaction with ERCs may result in new employment and internship opportunities for students, and even lead to the development of new research projects and thrusts for the ERC.
Many creative approaches have been developed to strengthen the link between industry and students in the ERC program and provide opportunities for industry to mentor students. Teams of students and faculty may travel to companies for presentations, meetings, and tours. Industry also may design projects or suggest problems for study by a team of students in the ERC. For instance, a team approach to industry problems is used at the University of Washington.
FEATURED EXAMPLE:
The Industry-Student Partners in Research (INSPIRE) program of the University of Florida’s ERC for Particle Science and Technology allows industry partners to propose an undergraduate project. If the project is accepted, undergraduate(s) given the project are provided with an industry mentor as well as an ERC faculty mentor. [60]
Industry mentorship is a powerful way to provide ERC students at all levels with a direct, apprenticeship-like experience with industrial practices and perspectives.
ii. Internships in Industry
The education program leaders believed that “One of the most valuable mechanisms of industry-education interaction is the student internship experience, in which the student is sent to the industry site. The student may be an undergraduate, an outreach student such as a Research Experiences for Undergraduate fellow, or a graduate student. The industrial internship formalizes industrial collaboration and ensures that the students’ education prepares them to contribute effectively upon graduation. The student gains from exposure to the real world of industrial product development and access to industrial researchers, and the company benefits from direct access to the student/technology inventor.“[61] However, over time, Preston remembers that when site visit teams would question students about their desire for internships, they would see many hands raised but when questioned about how many had actually participated in industrial internships, only a few hands would go up. When asked why, the general response was that their faculty mentors did not want them to leave campus to go to industry, despite obvious benefits, and perhaps because they were concerned that they would be “recruited” too soon before they finished their work on their Ph.D. theses and graduated.
CASE STUDY At the Rutgers University-based C-SOPS, industry mentors are integrated at the project level. Industry mentors are invited to co-mentor students and postdocs on all projects, and matches are facilitated by C-SOPS. Companies designate specific personnel to serve as mentors, with the number of mentors determined by the level of participation—Level 1 sponsors have several mentors; Level 2 sponsors are limited to two project mentors. Each project has multiple industrial mentors, with one serving as a lead mentor. Roles are clearly defined, including communication and progress standards. Mentors provide formal assessment of specific project progress at IAB meetings to focus on results and deliverables. Mentoring allows for input at the industry “grassroots” level within a company, while maintaining upper-level strategic involvement at the IAB level. Mentoring with the testbeds may play a critical role as these are closer to commercialization, and industry involvement may play a translational research-to-development role. There are distinct pluses, downsides, and challenges to this model. Pluses: The industry mentor has a vested interest in solving a process or manufacturing problem and technology partners are engaged, since the project is focused on their future product. This distributed model of industry engagement makes it more valuable to companies, as interactions are not limited to one person within the company (both high-level strategic and “grassroots” engineering support). Companies often have meetings to bring together all of their mentors participating in projects. The value of the overall engagement can be communicated to upper management, thus making participation in C-SOPS more tangible to upper management. Downsides: Creation and management of the mentor activities is very time-consuming. Discipline may lag at critical periods if teams have scheduling challenges. There tends to be more one-way communication from the center to industry, and this may not be as interactive as desired, since most of the team meetings are done via teleconferences due to restriction of industry travel. In some cases, certain industry personalities may dominate. Challenges: The IP protection process is challenging with outside mentors closely involved in projects. On the flip side, the industry mentors may be too close to what they are doing within their company, and may remove themselves from projects to protect the company’s interests or intellectual property. Best Practices Manual, Industrial Collaboration and Innovation Chapter 5, section 5.2.3.5. http://erc-assoc.org/best_practices/52-building-industrial-constituency> |
Nevertheless, the ERC Best Practices Manual advises that, “Industrial advisors of these interns commonly report innovative products, shortened development cycles, leveraging of industry resources, and joint research projects. This experience also has several benefits for the student. The relationship with an industry engineer provides a unique and essential educational experience. Work in an industrial environment provides the base for a student’s career in industry or academia, enables him/her to experience a team/systems approach to research and development, and cements professional relationships. This process also extends the influence of the ERC into the industrial sector.”[62]
iii. Industry Personnel in Residence
While the idea of industry personnel serving long-term residences at ERCs would surely benefit an ERC and the industrial personnel, this opportunity was not often pursued by industry personnel because some viewed such an absence to be a threat to their current positions in industry. There were some notable exceptions. One exception was the Center for Interfacial Engineering at the University of Minnesota, which established a scanning tunneling electronic microscope facility where industry personnel could be trained to use these (at the time new and revolutionary) microscopes. That brought many of the ERC’s industrial members on campus for extended stays, affording them not only facility with the new microscopy but also the time for joint research and deeper interaction with students. That ERC set up an Industrial Fellows program through which 20 ERC graduate students worked with industrial representatives from 19 different firms. At the Columbia University ERC more than 20 adjunct faculty from IBM, Bell Labs, Bellcore, and Phillips Labs routinely taught ERC-related courses in the affiliated departments.[63]
iv. Life-long Learning for Industry in ERCs
The ERC education leaders noted in their Best Practices Education Programs chapter that “As part of the lifelong learning aspect of their education programs, ERCs also sponsor seminars, workshops, and short courses to bring industry to the center (or take the ERC to industry), transfer technology and ERC research to industry, and encourage faculty exchanges with industry. Certificate programs (discussed in Section 4.4.3, “Curriculum Development”) are an increasingly useful way to bring industry to the ERC (and take the ERC to industry). Many ERCs use distance learning to link both multiple ERC institutions and industry partners (see Section 4.7, “Delivery Systems for Education Programs”)”.[64]
Lifelong learning, or continuing education, is an important outreach channel for ERC education programs—particularly significant in view of the interdisciplinary, industrially relevant research of ERCs and its goal of a diverse, multicultural workforce. It is not enough for an ERC to train students and send them out into industry and academia. The center’s mission also includes educating the public in the developing frontiers of science, engineering, and technology; retraining engineering and industrial workers in new technologies and research areas; and designing programs to reach new audiences with new engineering and technological innovations. Continuing education is central to many ERC industrial and education programs.
- For example, Clemson University’s Center for Advanced Engineering Fibers and Films offered short courses for industry personnel through continuing education programs, and the Packaging Research Center of Georgia Tech offered continuing education to employees of its industry partners.
Many ERCs develop short courses or workshops for industry, often co-taught by ERC faculty and industry.
- For instance, the ERC for Particle Science and Technology, at the University of Florida, typically offered four to six such short courses yearly.
- The Packaging Research Center offered two-week modularized courses every May, co-taught by faculty and industry.
- The Multidisciplinary Center for Earthquake Engineering Research at the University of Buffalo offered its Professional and Continuing Education (PACE) series of short courses for professionals, to inform them of advanced technologies in earthquake engineering.
Professional programs offer lifelong learning, particularly for career development and presenting ERC educational innovations to impact the young or established industrial engineer. These programs are attractive to industry as means of keeping their work forces up to date and minimizing retraining time loss. With constantly changing technology and current trends towards industrial downsizing, the professional engineer is more and more pressured to be aware of current and relevant research trends. Industry needs the best quality and most efficient continuing education possible, and ERCs are well positioned to deliver this service.
FEATURED EXAMPLE:
The Multidisciplinary Center for Earthquake Engineering Research (MCEER) offers a Professional and Continuing Education (PACE) program, which provides engineers with practical knowledge about current and emerging technologies and design procedures that will enhance their career skills, while improving the safety of the built environment from earthquakes and other hazards. Since MCEER has five participating universities, courses can be offered at locations around the nation. MCEER launched PACE in the fall of 1996 with a short course on Passive Energy Dissipation for Seismic/Wind Design and Retrofit, which was offered in Seattle, San Francisco, and the Los Angeles/Irvine area. The second course session was held in the Los Angeles/Irvine area, in February 1997. Past and potential course participants can subscribe to a mailing list for upcoming courses.[65]
ERC education programs, in conjunction with industry personnel, have developed certificate programs and distance learning models. Courses developed for university credit were offered as distance learning classes. The Best Practices Manual notes the following:
- For example, the ERC for Environmentally Benign Semiconductor Manufacturing at the University of Arizona offered a distance learning class in Microelectronics Manufacturing and the Environment in spring 2001, via the University’s distance learning program and the National Technological University (NTU).
- In 2002 a series of web-based modules, each on a single topic relevant to industry and taught by experts, is being piloted by the ERC for Particle Science and Technology in conjunction with the distance learning department of the university’s College of Engineering.
- The Georgia Tech/Emory Center for the Engineering of Living Tissues is working with the Center for Distance Learning and the Interactive Media Technology Center, both at Georgia Tech, to develop an internet course in tissue engineering for graduate students at other universities and for industry.
- The Packaging Research Center of Georgia Tech offers several web-based courses accessible worldwide, in a joint program with the IEEE Components, Packaging, and Manufacturing Technology Society.[66]
“The trend toward globalization and internationalization has affected ERC education programs, particularly in the industrial area. Many ERCs bring in visiting foreign scientists and engineers and provide mechanisms and assistance for this process. The now-graduated ERC for Net Shape Manufacturing at the Ohio State University offers a visiting scholar’s program for international engineering students, resulting in a master’s thesis on an industrially relevant research project, which is recognized by the student’s home institution. In working out international exchanges of students, faculty, or industry representatives, special planning is needed to manage issues such as immigration, visas and work permits, international travel, and security. The education coordinator/director may need to work with industrial liaison and administrative director on various aspects of this planning and coordination.”[67]
7-C(f) Lessons Learned from Evaluations and Reports
Preston and Parker commissioned the Stanford Research Institute (SRI) to carry out a study of the institutional impacts of ERCs on their home and partner institutions. The study included the 18 ERCs operating for ten years between 1990 and 2000—i.e., successful ERCs in the Classes of 1985 through 1990.
The overall findings regarding education were:
“Education was the area in which the most widely spread impacts of ERCs…. Although the effects of ERCs per se were often difficult to unravel from the many concurrent influences pressing for change in science and engineering education during the last decade, particularly at the undergraduate level, in every case but one at least some changes in the direction of increased interdisciplinary exposure, team-based research experience, industry interaction, and/or undergraduate involvement in research was at least in part attributed to the models set forth by the new curricula and courses, Research Experiences for Undergraduates (REU) programs, seminars and workshops, and other educational activities initiated by the ERCs. The changes attributable to the ERCs were most clearly evident in those departments with direct participation in the Centers, but were generally also apparent to at least some degree throughout the colleges of engineering. In some cases, the educational impacts of the ERCs were experienced as campus-wide phenomena, literally affecting practically all colleges and departments throughout the university. [68]
i. Curricular Impacts of ERCs
The framers of the ERC construct insisted on the involvement of undergraduates in ERC research programs throughout their academic year and an impact of current research on their courses in order to better prepare graduates for practice or further study. By the mid-1990s, the ERC Education Directors understood the difficulty of making impacts on the undergraduate curriculum in engineering since it reflected a community view of required knowledge to become an engineer. However, ERCs did have a significant impact on the curriculum in engineering and some lessons learned from their findings, published in the Highlights of Engineering Research Centers Programs, are summarized below:
- The impact is more of a trickle-down and more real than apparent from the course listings, since it is the ERC faculty who are bringing new knowledge drawn from the ERC cross-disciplinary culture and ERC testbeds into the classroom.
- ERC students are high quality and ready for engineering practice because of their exposure to team research and the opportunity to work with faculty and students from different disciplines, giving them a broader perspective.
- Through ERCs many undergraduates discover for the first time the excitement of research, resulting in a high percentage deciding to continue to graduate school.
- Team teaching was not prevalent before ERCs but ERCs made significant advances toward showing how and why courses should be taught jointly by different departments.
- The commitment of ERC faculty to teaching courses and educating students demonstrated that the ERCs were not just a research unit, but an integral part of the university and its mission.
- ERCs are a “neutral ground” offering a non-threating way to experiment with new types of courses and reduce duplication of courses across disciplinary departments.
- ERCs offer a contrast to the impersonal style of learning found in most undergraduates’ experience, offering personal interaction with faculty, other students, and industrial personnel, so that the ERC culture promotes effective training and the development of personal and professional confidence.
- Much of the cross-disciplinary fertilization that occurs in ERCs is sparked by the students themselves, who discover a common identity and esprit de corps with students from other disciplines with whom they would otherwise have little or no contact.
- ERCs serve as “missionaries” for science and technology through their outreach programs that reach students at the pre-college level and include students from institutions without strong research programs.
- An unexpected outcome is that, due to the ERCs’ integration of research and education, the cost of the education programs is relatively low, a benefit that is realized through the commitment of the ERC leaders and faculty to improvements in engineering education. [69]
The independent assessment of the institutional impact of ERCs carried out by Catherine Ailes and Erwin Feller corroborated these findings regarding curricular impacts of ERCs:
The impact of the ERCs on the courses and curricula offered by their host universities were substantial. Virtually all of the ERCs included in this study have created new courses and modified existing courses at both the undergraduate and graduate levels. Most of these courses were designed to reflect the interdisciplinary, systems-oriented research undertaken by the Center. Enrollment in these courses by students not otherwise directly exposed to the ERC often served as a multiplier of the number of students the ERC was able to influence directly. Courses developed by the ERCs generally involved enrollment of students from multiple departments, thus providing faculty as well as students with an opportunity to interact with students outside their home departments.
In a number of cases, company representatives were included in the Centers’ design of these new courses or course modifications, thus bringing industry’s thinking to bear on the content to which students would gain exposure. In some cases, industry representatives participate in Center-developed classes and symposia, either as lecturers or as students.
At a number of universities, the interdisciplinary courses developed by the ERCs were credited with leading to increased enrollment by students from one department in courses offered by another.
- At both MIT and Montana State, for example, engineering students, whether associated with the ERC or not, are increasingly enrolling in courses in biology. In 1993, MIT began requiring that undergraduate engineering students take at least some coursework in biology, which was attributed in part to the presence of the Bioprocessing ERC.
- At the University of Maryland, the new Project Gemstone, which reportedly stemmed largely from an attempt to emulate the observed educational accomplishments of the ERC, incoming students are placed in teams that cross all disciplines to work together throughout their college careers.
- The award-winning curricular changes introduced by the Carnegie Mellon Data Storage Systems Center in the electrical and computer engineering department were credited with leading to similar changes in the entire College of Engineering at CMU as well as in Engineering Colleges at other universities.
In addition to new or modified courses, many Centers have also developed or spurred the development of entire new degree programs. New degree programs are often cross-school, so courses are cross-listed, reflecting curricular changes broader than in engineering.
- The Maryland ERC, for example, developed a new M.S. in systems engineering, while the Purdue ERC has developed an M.S. option in manufacturing. As another example, the Mississippi State ERC developed a new M.S. and Ph.D. program in computational engineering and was also considered instrumental in the initiation by the University of a new Ph.D. program in mathematics and an M.F.A in electronic visual imaging.[70]
- At one institution, MIT, the Bioprocessing ERC was considered the catalyst for the creation by the University of a new multidisciplinary organizational structure within the College of Engineering to formalize the role of biology within the entire engineering curriculum. The new organizational unit will have the ability to hire and promote its own faculty, thus facilitating the hiring of truly interdisciplinary researchers and educators who might not be attracted or recruited by the more traditionally disciplinary bound departments. [71]
ii. Undergraduate Involvement in Research
The Ailes/Feller study had the following findings regarding the goal of the ERC Program to involve undergraduates in ERC research programs to better prepare them for industrial practice:
“The active involvement of undergraduate students in Center research activities is a requirement of the ERC Program. Each of the ERCs therefore has at least one program through which undergraduates from their own university participate in Center research. Programs for students within their own institutions generally entail students working in research laboratories under the direction of ERC faculty and/or graduate student mentors. The undergraduates often receive either course credit or stipends for their work. They sometimes use the Center-based research experience for a senior honors thesis or design project. Their involvement with the ERC generally provides these students not only with exposure to research per se, but also to faculty and students from other departments within the university and to industry representatives who are visiting or working at the Center.
Many ERCs also have summer REU programs in which undergraduates from other universities join undergraduates from the center institutions and participate in the Center’s research. Funds for REU students from non-ERC institutions can come from several sources. ERCs are allowed to compete for supplements to their base award to fund the involvement of undergraduates from these institutions who are women, underrepresented minorities, and persons with disabilities. REU support can also come in the form of separate awards made by the NSF REU Site Program. Finally, numerous ERCs use their own funds or obtain them from non-NSF sources to include additional undergraduates in REU activities.
The educational programs offered by the ERCs were generally considered a major attraction to industry. Students from these programs were not only viewed as better prepared for jobs in industry, but as having a better sense of what a career in industry was likely to be like.
In many cases, the ERC was directly credited with a major change in the university culture surrounding involvement of undergraduate students in research.
- At Montana State, for example, the ERC was said to have been the first effort on campus to bring undergraduates into the laboratories. The Center developed a system in which graduate students mentor the undergraduates in much the same way that the faculty mentor the graduate students. It was a high-profile part of the Center, and, in conjunction with other influences toward greater research experiences for undergraduates, has led to much more of this throughout the University. The University has now established a Coordinator of Undergraduate Research to facilitate undergraduate research experiences on the campus as a whole.
- At the Carnegie Mellon Data Storage Systems Center, relatively few undergraduates were involved as research assistants in the research activities of the Center’s predecessor research operation; once ERC funding was received, however, undergraduates were drawn into the research effort, and frequently ended up publishing articles with their graduate student and faculty mentors.
- In other cases, the ERC’s efforts at the undergraduate level were said to have come at a time where other forces on the campus were pushing in the direction of greater undergraduate research involvement, and to have reinforced or served as a model for programs or activities with related educational goals being established elsewhere in the university.
- In a few cases, however, the ERC itself was reportedly one of the few instances on the campus in which undergraduates had any real exposure to research and faculty in general remained resistant to the notion of involving undergraduates in their research in the belief that the degree of mentoring that would be required was more trouble than it was worth. [72]
iii. Educational Impacts of ERCs on Graduate Students
The Ailes/Feller study found that the ERC goals for an interdisciplinary, systems-focused education with significant impacts from industry were significantly met by ERCs, in contrast to most graduate educational experience:
In most fields of science and engineering, graduate students receive a significant amount of support from faculty research funds and, as they progress through their department’s degree program, they acquire increasing skills to become an important resource in the conduct of research. Typically, graduate research assistants work for individual researchers and move from relatively simple tasks, such as setting up apparatus or monitoring and collecting data from experiments, to taking on increasingly complex activities and responsibilities. Faculty mentorship usually takes place in the context of a departmental laboratory aimed at guiding the student toward a disciplinary degree. Student involvement in industrially sponsored research is often limited by the ability of projects to provide students with sufficiently complex problems to form the basis of a doctoral dissertation/thesis, and a graduate assistant’s interaction with industry is often limited to the representative(s) of the sponsoring firm.
By contrast, graduate student involvement in an ERC is unique is several respects.
- First is the degree of cross-disciplinary interaction and exposure.
While students typically
work under the supervision of an individual ERC faculty member,
- individual faculty are part of ERC research teams that generally involve faculty and their students from multiple departments.
- ERC students are integral parts of these teams, exposing them to the thinking and problem solving skills and expertise brought from disciplines other than their own fields of concentration.
- Second, ERC
students typically have considerably greater interaction with industry
than is the norm.
- Industry personnel often work in the Center as fellows or adjunct faculty members, sometimes serving as mentors for students or members of their dissertation/thesis committees.
- Students themselves often do internships or other research at company locations.
- Poster sessions at which students present their research are often held in conjunction with ERC industrial meetings, affording industry representatives an opportunity to meet with students and learn about their work.
- ERCs frequently conduct their own seminar series at which students discuss their research with industry representatives as well as faculty and peers, or at which industry representatives present lectures.
The ERCs were often credited with serving as a major attraction in the recruitment of high quality graduate students, especially within those departments most directly involved. In a few cases, the presence of an ERC on the campus was credited with playing a role in an increase in the quality of students enrolling in the college of engineering more broadly. Graduate students generally reacted extremely favorably to the interdisciplinary course work as well as the research exposure they obtained through association with the ERC, and most were enthusiastic about careers in industry.
Some, however, worried that the degree of interdisciplinary and problem- solving orientation of their ERC-associated educational experience might represent a disadvantage to their attempts to secure a position in academia, where the more traditional disciplinary orientation is far more common.[73]
In a comparison, with non-ERC institutions, Ailes and Feller found that, “Roughly
60% of ERC institutions reported that more than 50% of their faculty engage in significant interactions with industry, compared with 32% of non-ERC institutions that reported that degree of industry interaction.[74]
These assessments and evaluations are, overall, quite positive indicators of the impact of ERCs on undergraduate and graduate education; and they were corroborated by a SWOT analysis of ERC Education Programs carried out during the 1998 ERC Program Annual Meeting by the leaders of the ERC education programs. The participants developed a report, but it is marked confidential and cannot be included in this ERC Program History. Preston remembers that, while the SWOT generally confirmed the positive findings noted above, it did uncover tensions between the research and education efforts in ERCs that have been a longstanding part of the university culture. Those issues were based on: “rumblings” from some faculty that the ERC Program’s emphasis on education beyond graduate education took away from the strength of research efforts focused on graduate students; the fact that the university culture emphasizes individual efforts and rewards research output over educational output; the difficulty of integrating undergraduates into research projects; and the fact that pre-college education was often considered beyond (or beneath) the responsibility of universities.
The ERC Program understood those long-standing cultural pressures and developed systems to counter them; and the ERCs themselves developed new efforts that brought the two cultures together in course and curriculum innovations. The performance criteria that promoted the involvement of undergraduates in research was supported by the requirement that graduate students work in teams with the undergraduates to phase them into the research projects. Undergraduate and graduate students carried out most of the pre-college activities and for the most part enjoyed working with these young students, as in many cases they were not so far in age from themselves. In addition, they often remarked at site visits how much they would have enjoyed and valued such a program when they were pre-college students. It gave many of them a lot of satisfaction to know they were contributing to helping young students understand engineering and find a pathway to the field.
7-D Transforming the Model for the 21st Century: (Gen-2 & Gen 3): 2001–2014
7-D(a) Achievements of ERC Education Programs at the Start of the 21st Century
As noted above, the first and second generations of ERCs had developed education programs that produced graduates with the following traits/characteristics compared to those who received a non-ERC education. ERC students:
- have a broad cross‑disciplinary education and awareness
- have a broader outlook, integrate knowledge more readily
- work better in a collaborative mode
- have a more global perspective
- have more effective communication skills, both oral and written
- benefit from professional conferences and participation in the NSF review process
- have training in systems-level engineering research
- are more flexible in resolving research problems by using other disciplines in to help resolve problems
- have experience in interactions with industry
- pursue active involvement in team activities
- tend to seek more research-oriented jobs than non‑ERC graduates
- have a more interdisciplinary perspective on responsibilities
- need less on-the-job training and are able to contribute to real work earlier in their employment than most other graduates
- have hands-on experience and are willing to apply hands-on-skills
- are more sought after by industry, take responsibility and contribute earlier in their careers, and rise to positions of leadership more often.
These significant differences were noted by the authors of the 2002 edition of the Education Chapter of the ERC Best Practices Manual.[75] They point to the success of ERCs in achieving the culture change in engineers envisioned by the original goals for the ERC Program. These observations were corroborated by evaluations noted above.
Based on these achievements, in 2000 the ERC Program embarked on strengthening the Gen-2 ERC education programs further as the decade began, especially its pre-college component and the roles of ERC students as leaders in their ERCs.
7-D(b) Strengthening Gen-2 Pre-College Education Programs
The motivation for strengthening the pre-college programs was to broaden the horizons of young students regarding careers in engineering by educating their teachers about engineering and the engineering process.
i. Pre-college Outreach and Research Experiences for Teachers
At the start of the Millennium, pre-college outreach continued to be encouraged and new ways to join students and their teachers into ERC activities began to be more widespread across the ERCs.[76] By “expecting and encouraging” it through annual report guidelines, database reporting, and performance criteria, a number of innovative pre-college outreach programs emerged from the ERCs. These were the models for the requirement for the involvement of pre-college students and teachers in the FY 2002 ERC solicitation,[77] which created the Class of 2003.
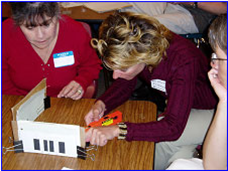
Figure 7-4: RET teachers at work on a summer ERC project |
The experimentation with involving pre-college teachers in ERCs began in 2001, at the request of the then Acting Assistant Director for Engineering, Louis Martin Vega. Preston and Mary Poats awarded supplemental support to two ERCs, the Center for Computer Integrated Surgical Systems at Johns Hopkins University (Class of 1998) and the Gordon Center for Subsurface Sensing and Imaging Systems at Northeastern University (Class of 2000), to demonstrate a pilot Research Experiences for Teachers(RET) Program (Figure 7-4). This program served as the model for the later-to-be-required RET programs in ERCs and, later, the Engineering Directorate’s RET Sites program. These two experiments demonstrated that pre-college teachers could effectively learn engineering concepts at these ERCs and develop course modules to bring engineering to their classrooms. As a result, by 2002 all ERCs were required to conduct RET programs. The success of these efforts was recognized by the Directorate for Engineering and Mary Poats was assigned to lead a Directorate-wide RET Sites program, which was cited approvingly by the National Science Board (NSB) in its later report, “Moving Forward to Improve Engineering Education.”[78] The NSB recommended that NSF expand the Directorate’s RET Sites program because of its impact on bringing engineering concepts to the K-12 classroom. The program was expanded from its start-up budget of $4.0M to its FY 2018 budget of $5.8M, encompassing the CISE Directorate as well as ENG. (From an internal NSF 2007 RET Site Program Assessment.[79]) The BIO Directorate has as a separate RET Sites program.
ii. Examples of Pre-College Education Outreach to Students and Teachers
Every ERC conducted a variety of education programs involving K-12 students and teachers. These ranged across the spectrum from involvement of ERC students and faculty in local science fairs to long-running programs offered in the schools, to bringing pre-college students on campus for research and education experiences. The common aims of all these programs were to spread awareness of what engineering is and what engineers do, to interest more young students in engineering and technology as a possible career, and to broaden the demographic diversity of the pool of potential engineers. Some examples follow from a 2008 summary of ERC education program achievements:[80]
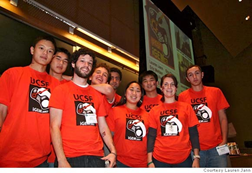
The winning Lincoln High School iGEM team fielded by SynBERC |
1. iGEM —The International Genetically Engineered Machine Competition (iGEM) is a design competition originally initiated by staff and faculty at MIT and integrated into the Synthetic Biology Engineering Research Center (SynBERC), which was based at the University of California, Berkeley. It is currently a foundation.[81] The annual competition teaches teams of students (mostly undergraduates, although high school teams are allowed to enter) how to design and execute a bioengineering design project over the summer, while applying the principles of synthetic biology. Student teams are given a kit of standardized biological parts, which they can use to build biological systems and operate them in living cells. At the end of the summer, teams gather at a host university to present the results of their work, interact with other research teams, and win prizes.
Of the 57 iGEM teams competing in Summer 2007, only two from the United States won prizes in the competition. One of them, from Abraham Lincoln High School in San Francisco, Synberc outreach affiliate, bested university teams from Harvard, MIT, Caltech, and Princeton to finish among the top six finalists worldwide. The high school students made an artificial organelle inside a cell, called a synthesome, which carries out various functions within the cell. The students used gene-splicing techniques to tag outer portions of their synthetic outer cell membrane with new chemical signals so that they wouldn’t be recognized and destroyed by other portions of the cell. The students learned to control the flow of information within the cell. They worked in the lab of Wendell Lim, a molecular biology professor at the University of California, San Francisco (UCSF), who was then Deputy Director of SynBERC, along with their mentor at Lincoln High, biotechnology teacher George Cachianes, who had worked in the biotechnology industry. All of the students on the Lincoln-UCSF team went on to attend public universities in California.
2. STAR Program —The Biomimetic MicroElectronic Systems (BMES) ERC at the University of Southern California had an extensive program of pre-college education built on the award-winning Science Technology and Research (STAR) program developed by Prof. Roberta Diaz Brinton, the ERC’s Education & Outreach Director. STAR I students had BMES faculty and student mentors who exposed them to science and engineering concepts starting in the third grade. STAR II students, in their later school years, came to the BMES laboratories to carry out research projects. In 2007 alone, 28 STAR II students were matched with 16 USC research laboratories, of which 11 were BMES laboratories. The students learned basic research concepts, designed their research projects, and prepared posters displaying their results. These students went on to pursue undergraduate degrees in engineering and biology at rates higher than their peers at their home schools.
3. Light and Optics Workshop— To make a lasting impact on K-12 student understanding of lasers, optics, and the physics of light, the Extreme Ultraviolet ERC for Science and Technology (EUV ERC), headquartered at Colorado State University (CSU), made a sustained, multi-year effort at Poudre High School in Fort Collins, CO, through the ERC’s High School Light and Optics Workshop. Every January, Poudre students—many of them Native Americans—attended 4 two-hour sessions held in the optics teaching lab on the CSU campus. Using optical equipment and lasers, the students worked through a series of experiments demonstrating fundamental concepts about the physics of light/optics and their applications. During the final workshop session, participants spent approximately two hours with Center graduate students as they toured the EUV labs to learn about current research projects and to hear from the ERC students about the excitement and challenges of careers in science and engineering. In 2007, 13 students (6 boys and 7 girls) participated.
4. “Content Institute” for Middle-school Teachers— The ERC for Collaborative Adaptive Sensing of the Atmosphere (CASA), headquartered at the University of Massachusetts, Amherst, held a week-long Content Institute to provide professional development for middle-school teachers. These were held each year at one of the partner universities of CASA. The Content Institutes were designed for middle-school teachers to deepen their knowledge of concepts and develop hands-on classroom activities with respect to the state science/ technology/ engineering and Earth and space science curriculum frameworks; become excited and informed about how engineering endeavors impact people and serve society; and consider methods of encouraging diverse groups of students to pursue engineering and science studies.
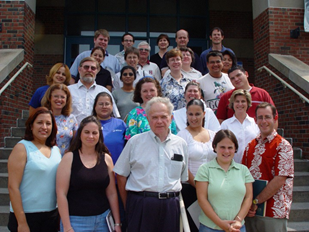
Middle school teachers at a CASA Content Institute |
In July 2007, 25 teachers from Kansas and Oklahoma met at the University of Oklahoma, in Norman OK, for the week-long Earthstorm Content Institute. CASA radar modules, field trips, and laboratories were integrated with the Earthstorm weather content. Over the course of the week, the teachers learned about electronics, meteorology, hydrology, and radar in the context of predicting severe weather events, and about the impacts on society of severe weather. In addition, hands-on workshop modules on using radar data in the classroom were implemented. The teachers visited numerous radar installations, including the CASA radars, the National Weather Radar Testbed, as well as mobile radar facilities such as the Shared Mobile Atmospheric Research and Teaching Radar (SMART-R). The teachers then spent time at the National Weather Service’s Norman office, the Storm Prediction Center, and the NOAA Radar Operations Center. This experience allowed each participant to see how radar data are collected, how these data are transmitted to users, and how radar information is visualized for presentation to the public.
In 2005, Preston pointed to the ERC education achievements shown in Figure 7-5 during her plenary briefing at the ERC Program Annual Meeting in the fall of that year.
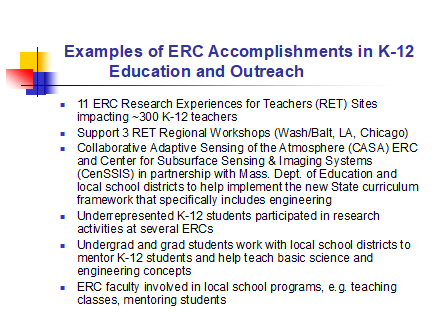
Figure 7-5: Example Achievements in Education and Outreach
7-D(c) Example Graduate and Undergraduate Educational Achievements of Gen-2 ERCs
i. Students Design Advanced Radar Network
An all-student team of 15 graduate and undergraduate students at the ERC for Collaborative Adaptive Sensing of the Atmosphere (CASA), headquartered at the University of Massachusetts, Amherst, spent much of their time during 2005 and 2006 creating an experimental radar testbed system, to be deployed in Puerto Rico, that aims to transform our ability to monitor heavy rainfall. Comprised of an array of miniature radar sensors, the system produces accurate rainfall data to be used to predict flooding and support other applications such as crop hydrology that require accurate rainfall estimates. The radar system became operational in 2007.
The student-run testbed system (see Figure 7-6), with graduate students leading the project team, offered both a unique research and educational experience. It required students to work across disciplinary and geographical boundaries and understand all facets of developing and implementing a testbed. The system covered a crucial 1.5 km-high gap in atmospheric weather-monitoring over western Puerto Rico that traditional radar technology cannot sense. The testbed also explored methods for more accurate measurement of rainfall and wind data than is now possible. One of many student design innovations was a system for allocating power-hungry activities (such as computation) to nodes with more reliable access to power, to minimize downtime.
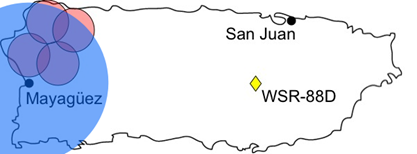
Figure 7-6: The student-run radar network consisted of two types of installations: a CASA “rooftop radar” (coverage shown by blue circle) located at the University of Puerto Rico-Mayaguez (UPRM); and CASA “off the grid” radars (pink circles) that were deployed at remote locations using solar panels to provide power. This array filled in a low-level coverage gap that the island’s only WSR-88D Doppler radar cannot “see.” This student project brought new insights to the faculty about how to handle distributed computation in the testbed and led to new research areas for the Center.
ii. Training for Bioscience Commercialization Through TI:GER Teams
To bring into the commercial market technical innovations that require skills from a variety of disciplines, the Georgia Tech/Emory Center (GTEC) for the Engineering of Living Tissues joined forces in 2005 with Dr. Marie Thursby, in the Georgia Tech College of Management, in a commercialization program called “Technological Innovation: Generating Economic Results” (TI:GER), https://www.scheller.gatech.edu/centers-initiatives/tiger/index. two-year TI:GER program educates graduate students in the challenges of commercializing new technologies and delivering innovative products to the marketplace by combining classroom instruction, team-based activities, and internship opportunities. Each TI:GER team is composed of a science or engineering Ph.D. candidate, a Georgia Tech MBA student, and an Emory Law student. In 2008 there were 9 bioscience-related TI:GER teams, 7 of which were formed around faculty inventors from GTEC. (See Figure 7-7) The program still continues as of the time of writing.
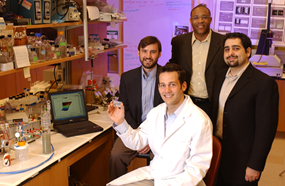
Figure 7-7: A GTEC TI:GER team displays their product. |
GTEC industry members have the opportunity to interact with a select group of TI:GER teams that give them short summary “pitches” of their projects. The advantages of this interaction with industry are: 1) the commercialization teams receive feedback from highly experienced industry partners; 2) the industry partners are exposed to development plans and progress in GTEC and bioscience innovations; and 3) industry members meet Ph.D., MBA, and JD students who could become valuable employees with industrially relevant experience.
7-D(d) Developing and Executing the Gen-3 ERC Education Construct
During the FY 2004 meeting of the Committee of Visitors to the Division of Engineering Education and Centers, Preston was challenged as follows: The ERC Program has been enormously successful in achieving its goals and broadly impacting industry and academe. However, the Committee challenged her to reposition the ERC program for continued success and impact over the next 20 years. As discussed in Chapter 3, Preston, the ERC team, and the Directorate embarked on an assessment of how the ERC Program might be repositioned to address the challenges of the 21st Century in other than the continuous-improvement approached used throughout the history of the Program. They drew on the sources shown in Figure 7-8.
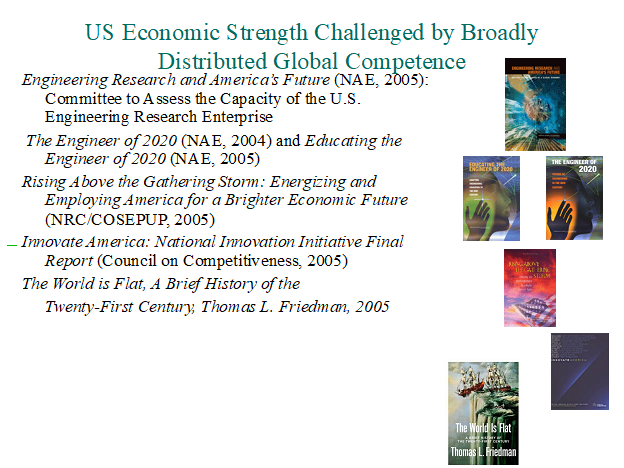
Figure 7-8: Calls to Action that Inspired a Repositioning of ERC Research and Education
In addition, they were inspired by remarks by Arden Bement, then the Director of NSF, to the National Science Board Education workshop at MIT on October 20, 2005:
- “Knowledge & human capital are key driving forces in global economy;
- Concentrations of S&T competence are now more broadly distributed globally;
- Industry buys commodity engineers and manufactures globally; therefore,
- US has to produce engineers who provide 4-5 times value-added through innovation.”
A major conclusion was that optimizing efficiency and product quality is not enough—we must optimize society for increased innovation. The outcome was a plan for Gen-3 ERCs that would have the following characteristics:
- Support a culture of innovation, a symbiotic relationship between research and commercialization, and life-long skill development;
- Stimulate diverse domestic and international talent to pursue engineering careers in the US;
- Impart the capacity to create and exploit knowledge for technological innovation.
- Carry out engineering research that leads in bridging discovery and technological innovation; and
- Produce engineering graduates who can function in a global world where design and production efforts cross national borders.
The resulting Gen-3 ERC solicitation (07-521)[82] retained the traditional ERC key features and, to deal with the need for an increased role in translational research and education to stimulate innovation, added new features to:
- Engage small innovative firms in ERC’s research teams;
- Engage ERC students in all phases of the innovation process;
- Build partnerships with academic and state and local government programs designed to stimulate entrepreneurship;
- Strategically design education programs to produce creative and innovative engineers;
- Provide cross-cultural, global research experiences through partnerships with foreign universities; and
- Build long-term sustained partnerships with a few pre-college institutions to infuse engineering concepts in the classroom.
These changes directly impacted the research programs and were integrated with changes in the education programs. Based on the experience of strategically designing research programs to achieve envisioned knowledge and technology advances, Gen-3 ERC education programs were to be more strategically designed to achieve the desired outcomes. To achieve this end, the Gen-3 ERC had to develop a design “hypothesis” for the desired characteristics of an ERC graduate and a strategic educational “design” to:
- Strategically nurture and develop graduates who are adaptive, creative innovators with the capacity to advance fundamental knowledge and exploit it to create innovations (new feature)[83]
- Structure the education program to develop graduates who have the knowledge, skills, and experiences needed to be successful in a globally connected, innovation-driven world (new feature);
- Include development of new course materials derived from ERC’s interdisciplinary and systems research, and if suitable, degree programs and options;
- Function with an education strategic plan that specifies desired characteristics, proposes how the education program will impart these to students, and how it will measure and assess progress and impacts through longitudinal data (new feature).
The new features of the pre-college education programs were based on an analysis of the features of the most successful ERC pre-college programs; those features for a Gen-3 ERC were:
- Form long-term partnerships with a small number of pre-college institutions (middle and high school) that are committed to infusing engineering concepts into the classroom (new feature)
- Develop materials and design experiences to include engineering concepts in pre-college education, with the goals of:
- increasing the enrollment of pre-college students in college-level engineering degree programs; and
- increasing the diversity of students interested in pursuing engineering education.
- Involve pre-college teachers in ERC’s research to enable them to develop course modules to bring engineering concepts to the pre-college classroom (an ERC RET Program within the core effort, not as a supplement)
- Offer promising high school students a “Young Scholars” research opportunity in the ERC’s laboratories (new feature, revived from the 1980s)
- Engage ERC faculty and students in pre-college activities as mentors and ensure that their efforts will be recognized and rewarded by their administrations (new feature).
i. Implementing the Gen-3 ERC Education Model: Operating with an Education Hypothesis to Ensure That Graduates Have ERC Characteristics
The first Gen-3 ERC Class was started in FY 2008. The proposal response, 143 preliminary preproposals in comparison to 109 for the last Gen-2 solicitation, was robust. It indicated that the engineering community was enthusiastic about breaking the new ground envisioned for the Gen-3 ERCs and building cultures that extended their research and education cultures to include innovation in partnership with industry, not just technology transfer to industry. Since these ERCs were to have foreign university partners, there was also an anticipation of the rich cultural and technical exchanges that would come from U.S. teams visiting their foreign partner universities and vice versa.
Shortly after the awards were made in the spring of 2008, teams of ERC staff and selected staff of ongoing ERCs made visits to each new center at its start-up to brief them on how to effectively start up and function as an ERC. They were briefed on the traditional features and the new Gen-3 features and advised that there was a large body of best practices for the traditional features, but they would be the pioneers in developing best practices for the new Gen-3 features.
The most challenging education feature turned out to be developing and implementing an education “hypothesis” that would function to strategically guide the experiences of ERC students so each would be assured to have a set of experiences designed to produce the desired ERC characteristics, such as the ability to bridge disciplines to solve problems and develop technology. In the past, evaluations showed that most ERC students had these experiences just from being immersed in an ERC culture; but there was a concern that it was not uniformly available to all ERC students, especially graduate students. The difficulty was compounded by the requirement that data on exposure to these experiences were to be collected and longitudinal assessments of progress were to be carried out. This meant that staff/faculty with education and education assessment experience had to be added to the ERC team. Extra funds were provided in Gen-3 ERC base budgets to support these efforts and other Gen-3 specific functions.
After the first set of annual reviews for the new Gen-3 ERCs and the first time they participated in the ERC Annual Meeting, it became clear that the word “education hypothesis” was not resonating with the engineering faculty or education staff. The idea of having an explicit design hypothesis to produce a particular type of student was alien to them even though they had been imbued in a culture that was designed to do just that—the single-investigator research engineer. The engineers began to understand that the motivation for this new approach to education was essentially an engineering design process applied to students instead of a piece of hardware. The faculty with education backgrounds that were brought into the ERCs had to learn engineering concepts, initially, and they had to develop a new reference to outcomes—not learning-specific outcomes but characteristics, such as team player, innovator, creative, integrating depth and breadth of knowledge, knowledge of industrial practices, etc. The education faculty/staff also had to learn that they had not just entered the ERC space to write papers that were based on the pedagogical theory of their fields, but that they had entered a new space with new intellectual challenges and new types of outcomes.
One of these education program leaders, Dr. Penny Jeffrey at the ERC for Future Renewable Electric Energy Delivery and Management (FREEDM) Systems, at North Carolina State University, decided to scope down the problem to a manageable scale by developing a portfolio of ERC experiences that all FREEDM graduate students had to have before they graduated.
The FREEDM Portfolio approach is illustrated in the following slides, presented at the FY 2012 ERC Annual Meeting (Figures 7-9, 7-10, and 7-11).
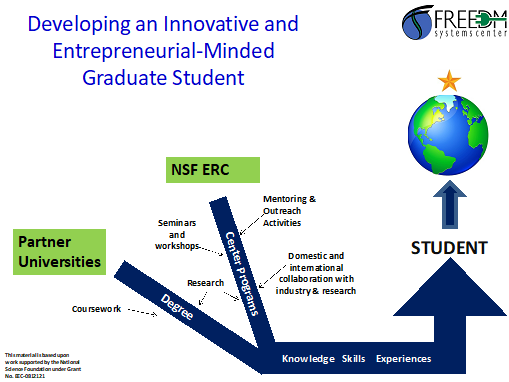
Figure 7-9: The FREEDM Graduate Education Portfolio
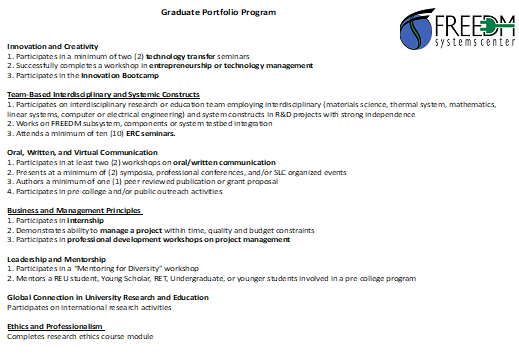
Figure 7-10: Elements of the FREEDM Portfolio
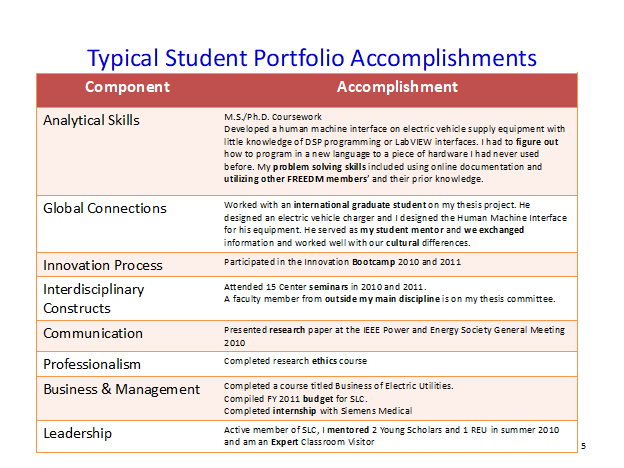
Figure 7-11: Sample Portfolio Accomplishments
Even with such a well-structured education program, at times implementing the program ran counter to the culture and Professor Jeffrey remarked as follows:
“With a strong level of support from an ERC Director and faculty with true appreciation for K-20 education and outreach, I feel the portfolio program experience is very worthy. Enabling ERC students to be involved in other areas of professional development and outreach beyond their student courses and research enables them to grow holistically.
However, in some ERCs I suspect the on-going struggle to
“prove” the importance and value for the student experiences to the
ERC Director and faculty could be daunting and exhausting, even with NSF’s
requirements.
Having a doctorate in education (knowing the theory, research, pedagogy of
education) is not the same as having a doctorate in just engineering. Yes,
there are some wonderful teaching[engineering] professors who value the true
learning process but most are researchers that are assigned to teach without any
true understanding of what that means and entails. Teaching is not lecturing.
And, I feel not enabling your student researcher to expand beyond engineering
coursework because you want them to only focus on the engineering research
product is not beneficial to their overall social and emotional well-being,
work productivity, and connection to how their community needs them.”
In time, the ERC Program dropped the term “education hypothesis” and replaced it with “desired skill sets,” which seemed to communicate the idea more effectively. Below are two more examples of how Gen-3 ERCs in addition to FREEDM developed their education programs in response.
CASE STUDY:
The ERC for Ultra-wide Area Resilient Electric Energy Transmission Networks (CURENT) at the University of Tennessee named their graduate education program “Innovation, Mentorship, Professionalism, Adaptation, Creativity and Technology (IMPACT).” CURENT developed recommendations for the skill sets ERC graduates will need to succeed in energy-related industries. CURENT ERC graduates will be grounded in the fundamentals of the Center’s education program and will develop creative characteristics if they are trained to have the following traits: analytical thinking, problem-solving attitude, entrepreneurial bent, is a leader and a mentor, and communicates effectively, behaves professionally, and demonstrates interdisciplinary and global views. The IMPACT program is CURENT’s strategy for producing a new generation of engineering graduate students with these desired characteristics to become creative, adaptive, and innovative. All students participating in the IMPACT program receive a personal online plan with detailed components to be completed and are expected to regularly meet with the education director or coordinator. For example, students are expected to gain experience working in a team-based research environment, be exposed to professional development opportunities and industry connectivity training, be required to complete an entrepreneurship or technology management course, show professional conduct, complete an engineering ethics course, and participate in education outreach activities.[84]
CASE STUDY:
The Nanosystems ERC for Advanced Self-powered Systems of Integrated Sensors and Technologies (ASSIST) at North Carolina State University developed the Translational Engineering Skills Program (TESP) Program. TESP focuses on teaching specific skills and attributes that graduate students need to become creative, adaptive, and innovative engineers with the ability to translate fundamental research into products and systems. These translational and professional skills enhance their ability to use their knowledge of medical devices and systems in the real world. They are clustered into “skill blocks” that include: systems thinking, entrepreneurship, industry/manufacturing, mentoring and leadership, communication, diversity awareness, and engineering ethics. Each of these skills is taught through a series of activities that are developed by the education directors with significant contribution from our technical Principal Investigators (PIs), industry liaison officer, medical director, and assessment coordinator. Some examples of activities are: a) invention disclosure workshop, patent mining session, and market research projects to improve entrepreneurship skills; b) mock journal reviews to help with students’ written communication skills; c) ASSIST mind mapping activity to improve students’ systems thinking and understanding of the center’s vision; and d) internships and industry visits that increase understanding of industry and manufacturing practices. The program requires successful completion of ten activities from a variety of skill blocks. The program and its activities were designed by the education directors in collaboration with our technical, industry liaison, medical, and assessment personnel, as well as other experts in the field. The education directors at all partnering institutions are responsible for assuring that activities are scheduled regularly, administering activities and assessments, and meeting with students twice per semester to monitor their participation in the program[85]
From these and other examples found in the ERC Best Practices Manual, it is clear that the challenge to ERCs to develop education programs strategically planned to produce graduates who would be more effective leaders in innovation in industry did result in programs carefully and purposefully designed to achieve those goals. Whether the desired outcomes were achieved awaits an independent evaluation of graduates and their supervisors.
ii. Gen-3 ERC Curriculum Development Impacts
ERCs continued to be required to integrate their research findings into the engineering curriculum, which was addressed through new courses and course modules and in some cases through wholly new degree programs. For example, the ERC for Revolutionizing Metallic Biomaterials (RMB) at the North Carolina A&T State University (NCAT), an HBCU, in partnership with the University of Pittsburg, developed the first Bioengineering BS and MS degree programs to be implemented at an HBCU. (See case study.)[86]
CASE STUDY: New Degrees in Bioengineering As part of its education/outreach initiatives, the ERC-RMB in 2009 initiated planning and implementation of MS and BS programs in Bioengineering. This included obtaining university and UNC-system-level approval for the programs and recruitment of faculty. ERC-RMB partner institution the University of Pittsburgh (Pitt) provided knowledge and shared resources in curriculum and lab development. Enrolling their first students in 2010 and 2011, respectively, the programs have grown their enrollments steadily. After graduation of their first cohort of BS students, the Biomedical Engineering (BMEN) faculty hosted an ABET accreditation visit in the 2015-16 academic year. The curriculum was modified and enhanced based on ABET feedback, following which the ABET sentNCAT an official letter of accreditation in Fall 2016 for the BS BMEN program, retroactive to October 2013. Now, in addition to having the nation’s first seamless BS-MS BMEN program offerings at an HBCU, NCAT is the first HBCU to have an ABET-accredited BS program in bioengineering. What is the impact? The bioengineering education programs at NCAT spearheaded by ERC-RMB faculty, are providing students with educational and research experiences in bioengineering that will instill in them a lifelong sense of learning, social responsibility, and commitment to improving the quality of life for all people. The BS and MS Bioengineering programs are continuously attracting a truly interdisciplinary student body, with current students drawn from across the engineering and sciences, from all over the nation. These activities are serving as a human resource pipeline, with BS and MS graduates proceeding to industry, medical school, and graduate research programs on a national level. The strong diversity of the programs is reflected in the demographic data, with a high level of enrollment of US nationals (~100% @ BS level, ~90% at MS level), high gender diversity (~60% female at BS level, ~70% at MS level) and underrepresented minority participation (~80-90% African-American in the two programs). http://erc.ncat.edu/?article=253 |
iii. Implementing the Gen-3 ERC Education Model in Pre-College Education
1. Activities to Stimulate Student Interest in Engineering.
Several ERCs developed games and other activities for pre-college students to stimulate interest in engineering. These were developed by teams of graduate and undergraduate students. Guy Simplant, described above, is one example from a Gen-2 ERC. A Gen-3 ERC example is WrestleBrania, developed by the Center for Sensorimotor Neural Engineering (CSNE) at the University of Washington.
Figure 7-12 shows a pre-college student wrestling with an artificial hand merely by clenching his fist and moving his arm.
CASE STUDY: In 2012, the CSNE launched the Tech Sandbox Competition, a team activity to develop new student projects using ERC-based research and equipment. The winning project, WrestleBrainia3000 (WB3K), has proved to be an engaging outreach tool and one of the CSNE’s greatest education assets. Since its creation, WB3K has been used to reach thousands of students at dozens of informal science outreach events including Brain Awareness Week, University of Washington Engineering Discovery Days, and Life Sciences Research Weekend. WB3K was also selected to represent the ERC program and the NSF at The National Science Fair in Dulles, VA, and the USA Science and Engineering Festival in Washington DC, and was a success at the 2014 Consumer Electronics Show. |
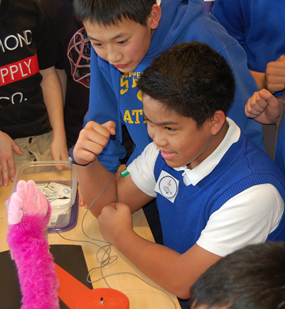
Figure 7-12: The CSNE WrestleBrainia game was a hit with young students.
2. Research Experiences for Teachers and Sustained Partnerships in Pre-college Education.
The major Gen-3 change in pre-college education involved developing long-term partnerships between the ERC and a small number of pre-college institutions, so that the teachers, faculty, and students from both sides could build a relationship in engineering education over time to give the pre-college students a view of a pathway to engineering education. In addition, there was a clear requirement that ERC faculty and students become engaged in these programs to serve as role models and mentors for these young students.
Pre-college education was often viewed as a “stretch” for engineering faculty, outside of their traditional mission. Some did not participate and some did so enthusiastically. A model for that type of effort was the STAR program at USC, which was discussed in Section 7-D(b)ii. When the STAR program became the key pre-college education program of BMES, it shifted its focus to biomedical educational experiences. Students and faculty from the BMES ERC were frequent visitors to the pre-college classrooms near the USC campus and the students, as they grew older, carried out research projects, at their level, on campus. Mark Humayun, the Director of BMES and Ted Berger, a thrust leader, were greeted at the middle school like rock stars, when they came on campus for the first time, as the students had first come to know them through online videos between the classroom and the USC campus.
All the Gen-3 ERCs were required to mount a self-paid RET program. One ERC not only had an RET program but also developed a model curriculum. Professor Ken Conner, the Education Director of the Smart Lighting ERC at Rensselaer Polytechnic Institute (RPI) (now Lighting Enabled Systems & Applications, or LESA) developed a pre-college curricular module that teachers could use to introduce lighting concepts and engineering to students. It’s called the Illumineer Curriculum.[87]
CASE STUDY: Smart Lighting, possibly even more so than the topics addressed at other ERCs, is very broadly multidisciplinary and involves background not generally found in any traditional STEM disciplines. Examples include lighting and other types of building design, human physiology and health, economics and business, etc. Our industry partners, especially, were finding that students graduating from excellent universities were not even conversant with the basics of lighting and those that knew something about lighting design (e.g., from schools of architecture) knew nothing of the science and engineering of solid state lighting. To better understand what is needed we defined a new discipline we call Illumineering, to differentiate it from traditional Illumination Engineering. usefulness is not tied to any particular delivery approach. We began the development of the Illumineer Curriculum by holding informal discussions with industry partners and then shared the ideas identified with all of our constituencies. The first versions of the curriculum matrix were produced collaboratively by the ERC Director Bob Karlicek and the Education Director, Ken Connor. Feedback on the matrix is obtained annually at our industry meeting, our faculty-staff-student planning retreat, etc., and continuously from everyone using it to guide the development of education and outreach content. One of the key steps in any of our programs is the identification of the aspects of the matrix included. Mentor training and mentoring activities are also formally included in all programs, with the present exception of those at the middle and elementary school levels. However, even the K-8 students are given information and tools they can share with their friends and family. They are not told explicitly of their mentoring opportunities but rather are empowered to show others what they have learned. http://erc-assoc.org/content/appendix-43-precollege-and-community-engagement-examples (4.3.3.1) |
Model RET programs were also developed. BMES continued its leadership of RET programs by developing the next-generation effort. Gigi Ragusa, a Professor of Education took the lead.
CASE STUDY: At the BMES ERC we conducted both a research experience for teachers (RET) program and a second teacher professional development program in middle schools across an entire district, using an associated grant from the California State Department of Education. The RET program was a 5-6 week summer program with teachers from middle and high schools and community colleges, while the second teacher professional development program (using Department of Education funding through the California Postsecondary Education Program, Improving Teacher Quality Program, or CPEC ITQ) was a middle school teacher and student program that included 53 teachers and 2,653 students. This second program included a 2-week summer program and monthly follow-up. Both programs had lesson study as a primary teacher professional development follow-up component and both programs targeted (a) teacher and student experimentation and inquiry via immersion in lab experiences, (b) science literacy for students, and (c) science motivation, interest, and engagement for students.[88] |
The RET effort had a series of outcome metrics for all levels of effort. These included a teacher instructional performance measure; a science teaching efficacy measure; a student science concept inventory; student science literacy measure; and a student science motivation, engagement, and interest measure. A full description can be found in the Best Practices Manual, Appendix 4-3.
The Synthetic Biology ERC, headquartered at the University of California, Berkeley, developed BioEngineering RET (BERET).
CASE STUDY: BERET focuses on the intersection between engineering, science, and mathematics through the interdisciplinary lens of synthetic biology. The program places in-service and pre-service teacher teams within faculty research laboratories and guides them to develop curricula based on their research that is relevant to the subjects that they teach in school and aligned with the educational standards for the subject addressed. BERET is jointly run by SynBERC and the Cal Teach science and mathematics teacher education program. The program has provided training for 52 teachers over a 3- year period. In-service teachers are middle and high school teachers who teach in diverse urban schools and have at least 3 years of teaching experience. Pre-service teachers are UC Berkeley undergraduates enrolled in Cal Teach, an undergraduate teacher development/training program. As follow-up to the summer, pre-service teachers teach their approximately 1-week curricular unit in the in-service teacher’s classroom.[89] |
The Center for Integrated Access Networks (CIAN) and the Department of Chemistry at the University of Arizona developed an RET program to bring teachers from pre-college schools serving predominantly Native American students to the ERC to learn about optics and chemistry. It’s called Research in Optics for K-14 Educators and Teachers (ROKET).
CASE STUDY: The ROKET target audience is teachers who teach to predominantly Native American K-14 students. Teachers spend 6 weeks at the University of Arizona performing research in optics and chemistry research labs. Each participant engages in a distinct “miniproject” which is carried out in their assigned lab with the help of a graduate student mentor and faculty mentor. In addition to the research experience, ROKET teachers complete (and receive 3 units of continuing education for) a 4-week course with the American Indian Language Development Institute (AILDI). The course focuses on methods for integrating traditional ecological knowledge with modern science curriculum. These two dimensions (research lab and AILDI course) work together to excite teachers about STEM research as well as provide the instructional tools needed to impart this excitement to their students in the context of Native American culture, thus bringing more Native American students to college in pursuit of STEM degrees. In 2013, the Optics Research Workshop (ORW), a new component of the ROKET program, was implemented in an effort to bridge the gap between the K-14 classroom and University of Arizona research labs in a culturally competent way. In the ORW, training with pertinent research equipment occurs, as well as lessons and hands-on projects that assist teachers in understanding symbiotic relationships that exist between optics/STEM and Native American cultures and communities. This is an integral component that provides teachers of Native American youth an understanding and tools to help their students make similar connections.[90] |
You can view below an article attesting to the impact of this program on a high school teacher, Dan Moreno, from the Menominee Indian High School in Keshena, Wisconsin, and more easily read the text (see Figure 7-13) on the following site https://www.optics.arizona.edu/sites/optics.arizona.edu/files/pdf/MIHS-Teacher-RiO-Article.pdf.
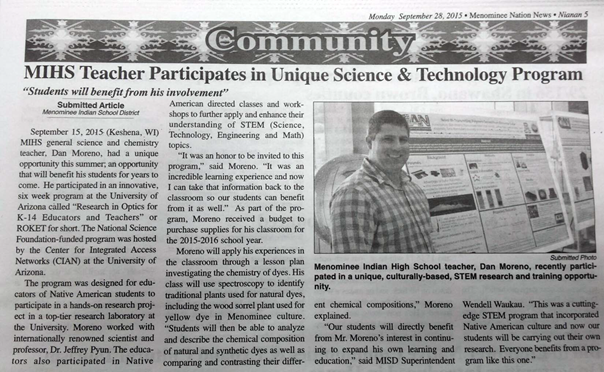
Figure 7-13: The CIAN ERC in Arizona developed an RET program aimed at Native Americans.
Sustained partnerships with pre-college school systems is a component of the Gen-3 education programs and the Center for Biorenewable Chemicals (CBIRC) at the University of Iowa has one of the best partnerships, with the Des Moines Public Schools District (DSMPSD), the largest district in Iowa.
CASE STUDY: The partnership with DSMPSD began in 2009, and CBiRC offers several summer professional development programs. Each program varies in length (from one to seven weeks) depending on the content. Additional support is provided by working with district professional development (PD) trainings during the academic school year. Another PD program, Symbi (Iowa’s GK-12 program), provides teachers with PD throughout the school year. Background: A needs assessment survey was administered when CBiRC was established. Based on the results of this survey, CBiRC offered to help middle school and high school science departments become better aligned between grades and schools. CBiRC provided support to create professional learning communities (PLC) for STEM teachers within the schools and across the district. A pilot PLC was created for the science staff in an adjoining high school and middle school building. CBiRC also began to implement PD programs for all middle and high school science teachers as well as elementary school teachers. The emphasis of the PD programs is biorenewables (both fuel and materials), the bioeconomy in Iowa, and “green collar” careers. The PD programs were designed not only to provide STEM content but also to train teachers to modify their curriculum to become inquiry-based, and to include more opportunities for student critical thinking. and problem-solving. Finally, CBiRC implemented a GK-12 program in Des Moines middle and high schools. This was a critical addition as it provides students and teachers with weekly interactions with professional scientists and engineers throughout the school year.[91] |
As impressive as these precollege education efforts were in the Gen-3 ERCs, many of the Gen-2 ERCs were still skeptical about the ERC Program’s decision to require the inclusion of pre-college in all Gen-3 ERCs. The 2007 ERC Program Annual Meeting was devoted to gaining input from the ongoing Gen-2 ERCs about the features of the Gen-3 ERCs to help with future program improvement. (See Chapter 3, Section 3-C(a).) It should be noted that these ERCs were not asked to participate in such an exercise prior to the release of the Gen-3 program solicitation, as it might have given those who might have been planning to compete for a second life cycle of ERC funding a competitive advantage over other competitors. The focus group of education program leaders favored the inclusion of pre-college programs in ERCs, but the overall recommendation based on input from all key-feature focus groups was less enthusiastic:
Participants agreed that there is significant value for engineering and for the nation in K-12 outreach and the majority viewpoint is that this key feature should be retained. However, a number of participants identified aspects of this key feature as the single most significant weakness of the ERC program, and more breakout groups recommended deleting it than was the case for any other ERC key feature. Participants questioned whether all ERCs have sufficient expertise to design and deliver effective K-12 outreach programs. Moreover, they felt that mandating educational outreach without providing significant resources to carry out these programs, as is the current situation, is not a workable approach. They had the following recommendations and observations:
- Rather than be solely responsible for these programs, ERCs should collaborate with successful, established non-ERC K-12 programs and/or with technical education specialists with K-12 expertise;
- Development of a realistic strategy for scaling up the K-12 impact of ERCs should be an important ERC program goal. Data should be collected, reported, and fed back to inform future program development. NSF should provide templates, expectations, etc., to the ERC faculty.
- Many participants felt that, given the lack of clear goals and limited funding available for these activities, the impact is small and tends to dilute the other activities and resources of the ERCs. In any case the impact is difficult to measure.[92]
Pre-college continued to be included in ERCs throughout the Gen-3 period. However, these issues raised were considered by the NSF working groups that were designing the Gen-4 ERC construct after Preston retired from NSF.
iv. Strategies for Instilling Leadership-in-Innovation Characteristics in ERC Students
In addition to the new features of Gen-3 ERCs, all ERCs were focused on producing ERC graduates with strong communication and leadership skills and (for Gen-3 ERCs only) skills that would prepare them to be successful innovators in a global economy.
1. Perfect Pitch Contests. In her role as coordinator of the Industrial Liaison Officers, Deborah Jackson (NSF) and the group of ERC ILOs often discussed education efforts to prepare ERC graduates for industrial practice. One of those ILOs who had worked in industry, Silvia Mioc, from the RPI Smart Lighting ERC, found that most engineering students still didn’t know how to briefly and succinctly explain their research goal and its impact. In 2011, Silvia, Deborah, and the ILO team came up with the idea of holding a contest among the ERCs to focus students on brief, 90-second descriptions of a project and its potential impacts.
Initially they called it the Elevator Pitch, after the idea that would serve as the basis for a start-up firm that might be pitched to a Venture Capitalist (VC) clearly and concisely in 90 seconds. By 2012, it was called the Perfect Pitch Contest because the goals for the pitch are broader than communicating ideas related to a potential start-up. The ILOs and their education program leaders organized the students during the summer to compete internally to represent their ERC at the upcoming ERC Program Annual Meeting in the fall, where the final contest would be held. Winners from the local level competed at the meeting and judges from industry selected the top three winners. Generous checks were awarded to those three and the first-place winner received the NSF ERC Perfect Pitch Trophy. The winner’s name and his/her ERC were inscribed on the trophy and it was sent to the winning ERC to keep for a year until the next competition. Bob Bower, the ILO from the EUV ERC, recommended that it be called the Lynn Preston Perfect Pitch Contest, to honor her role in championing both industrial collaboration and education in ERCs since the inception of the ERC Program.
Initially the competition was held alongside other sessions at the meeting, but the full group of ERC leaders asked that it be moved to a plenary session so they could view all the competitors and cheer on their own ERC’s competitor. Table 7-2 lists the winners from 2012 to 2017.
Figure 7-14 shows Aiva Ievins, from the Center for Sensorimotor Neural Engineering at the University of Washington, with NSF officials Tom Peterson (AD/ENG), Lynn Preston, and Deborah Jackson. Aiva placed first in the 2012 competition for her pitch to develop a neurochip to bypass an injured spinal cord to restore neurocontrol after injuries.[93]
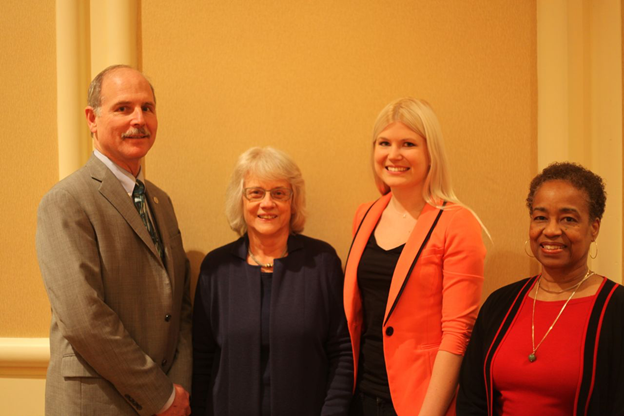
Figure 7-14: From left, Tom Peterson, then the NSF Assistant Director for Engineering; Lynn Preston; 2012 Perfect Pitch winner Aiva Ievins from the Center for Sensorimotor Neural Engineering at the University of Washington; and Deborah Jackson.
Table 7-2: ERC Program-wide Perfect Pitch Winners, 2011–2017
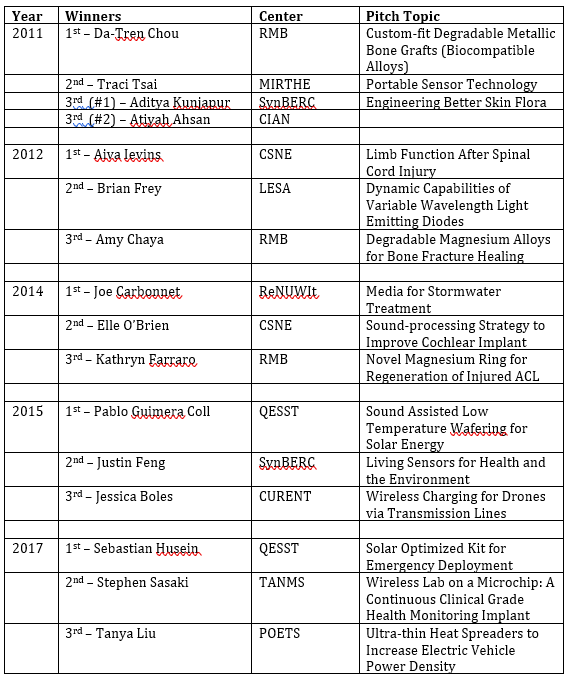
Figure 7-15 shows the 2017 first place winner, Sebastian Husein, with the trophy and a replica of his winner’s check. His pitch to potential investors was for an inflatable, floatable solar cell for use after hurricanes or other severe storms—an invention that was motivated by Hurricanes Irma and Maria. Sebastian was then a doctoral candidate at the Quantum Energy and Sustainable Solar Technologies (QESST) ERC at Arizona State University.[94]
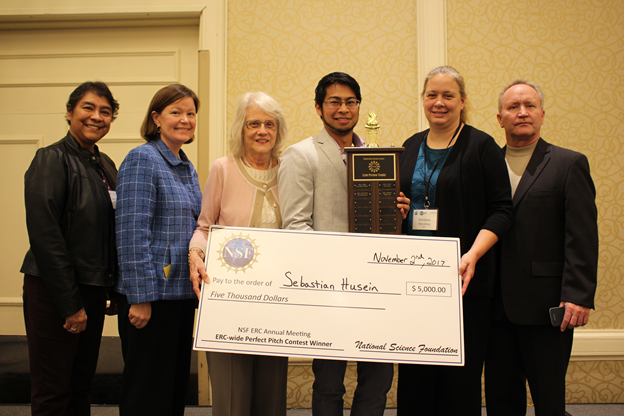
Figure 7-15: The 2017 ERC Program Perfect Pitch winner Sebastian Husein. From the left are Delia Saenz, the QESST ERC’s Diversity Program Director; Dawn Tilbury, NSF’s Assistant Director for Engineering (who formerly led testbed development at the University of Michigan’s Reconfigurable Manufacturing ERC); Lynn Preston; Sebastian Husein; QESST Center Director Christiana Honsberg; and John Mitchell, the QESST ILO.
2. Leadership Opportunities for ERC Students: The Student Leadership Councils. During this period, the ERC Student Leadership Councils (SLC) were established at all ERCs. They gave students the opportunity to view their ERCs as organizations, to lead teams of students in assessing the effectiveness of their ERCs, and to organize their ERC students into a community. These included:
- Involvement with strategic planning
- Annual SWOT (Strengths, Weaknesses, Opportunities, and Threats) analysis of the ERC by students as the ERC’s “customers” and workforce
- Participation in interdisciplinary teams of undergraduates and graduates with industrial association
- Mentoring other students
- Leadership, communication, and networking skills
- Self-organizing and self-education skills
- Organizing student social events and retreats.
Because the SLC members annually shared their best practices with each other in retreats at successive ERC Program Annual Meetings, they developed into a community of SLC leaders. As a result, they wrote a chapter for the ERC Best Practices Manual, which can be found online at http://erc-assoc.org/content/chapter-8-student-leadership-councils .
3. Incubator Programs to Stimulate Innovation. To give students and faculty more opportunity to experiment with advancing technology beyond the proof-of-concept phase, some ERCs developed incubators and other efforts to enable them to take their ideas one step closer to product. One of these ERCs, the Quality of Life Technologies (QoLT) at Carnegie Mellon University and the University of Pittsburg, developed the QoLT Foundry in 2008. It was directed by Curt Stone, a veteran entrepreneur who was an executive-in-residence at Carnegie Mellon. Support for this effort came from the base budget of the ERC, CMU, and an ERC Program Translational Research Award of $1.5M in 2009 (see sidebar).
THE ARRA and TRANSLATIONAL RESEARCH As a result of the negative impact on the economy from the “Great Recession” of 2007-2009, President Obama’s administration provided funds to government agencies through the Executive Budget to stimulate the economy. While the Director of NSF precluded centers from receiving the American Recovery and Reinvestment Act (ARRA) stimulus funding directly, that did not prevent the Assistant Director for Engineering, Thomas Peterson, from using funds “freed up” from other programs that did receive stimulus funding to provide the ERC Program with $9.0M. The Program chose to use some of those funds to support translational research efforts to stimulate new business development. |
As shown in Figure 7-16, the goal of the Foundry was to act as a venture capitalist providing funding to support the early phases of product description, business plans for advancing the technology, and early support for the startup of the spin-off firms.[95] Through this process, students became engaged in identifying and presenting potential technology commercialization opportunities. They also understood better the process by which the creation of spin-off companies can accelerate the transfer of the ERC’s technology to market because of the emerging trend throughout all industry sectors for established firms to make strategic acquisitions of small companies, rather than licensing technology at the completion of the research phase. They also found that technologies aimed to overcome specific impairments, of the kind on which QoLT focused, very often appeal to broader markets if positioned properly to emphasize their potential for enhancement and benefit to everyone. The students and their post-doc/faculty mentors used this understanding to grow the overall market for QoLT technologies by expanding the market beyond just the elderly and persons with disabilities. Their goal was for the QoLT Center to be the hub of an emerging QoLT industry sector that is comprised of established and start-up companies.
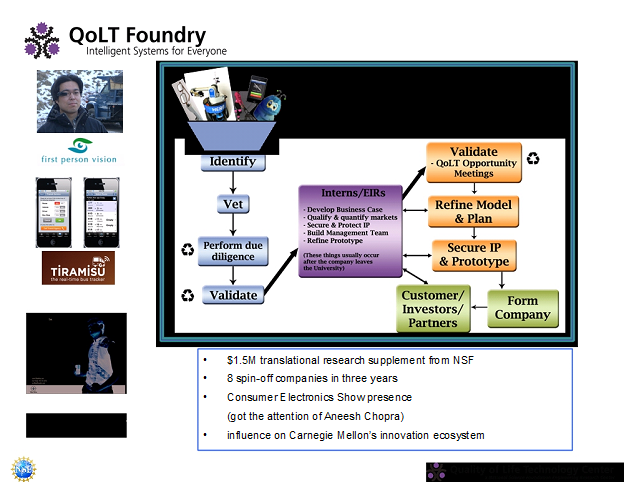
Figure 7-16: The QoLT Foundry was established to encourage and support entrepreneurship of the ERC’s students.
Not only was involvement in the Foundry beneficial to ERC engineering students, students from the CMU School of Business and Duquesne University also benefitted in new ways, as described in the following article excerpt:
Sam Yoosuk Kim, an MBA student at the Tepper School of Business, and Jen Sung, a rising CMU senior who is a double major in economics and decision sciences, are current participants — along with David King, a law degree candidate at Duquesne University. “I have learned what it takes to transform an idea into a viable company,” King said. “So many people have great ideas, but only a few are able to impact the world with their work.” The Opportunity Meetings feature lively debates on the market challenges for each presented opportunity and provide students — who may go on to lead QoLT companies — with suggested areas for further investigation. Thirty-five unique business opportunities have been presented and more than 250 people have participated in the meetings since their introduction in 2008.[96]
In September 2010, the Mid-InfraRed Technologies for Health and the Environment (MIRTHE) ERC, headquartered at Princeton University, worked with regional venture capitalists to create an Investment Focus Group (IFG) to introduce investment and industry professionals to emerging mid-infrared technologies and application opportunities. Joseph Montemarano, the center’s Executive Director, said at the time, “At this pivotal moment in MIRTHE’s growth trajectory, where increasing efforts are being placed on connecting academic research and the industries in which the technologies can be deployed, the Investment Focus Group has been created to provide guidance during the transition from R&D to realizing new profitable opportunities.” Bernadeta Wysocka, MIRTHE’s industrial liaison, added: “In the last four years the center has successfully developed its industrial membership program, integrating strong partnerships across a broad spectrum of commercial applications. Adding the technology investment community to the mix supports taking our technologies from test-beds to the marketplace.” Representatives from industry, financing, and entrepreneurship comprised the Investment Focus Group. The group’s purpose was to provide MIRTHE faculty and students with insight into how products and systems navigate the competitive marketplace.
4. Global Experiences Exemplar—NCAT’s partnership with Germany. One of the Gen-3 key features that gave Preston the most concern was the requirement that these new ERCs have foreign partner universities who would share in the research and education missions of the center. This concern was rooted in the origins of the program and its mission to strengthen U.S. competitiveness and the impact of decades of open sharing of ideas across national boundaries that benefitted scientific progress, but may have been detrimental to U.S. firms that moved more slowly on commercialization of innovations. In addition, over time many U.S. based firms had a significant investment in manufacturing and even research labs in other countries. The premise of the Gen-3 construct was that the foreign partner feature would provide opportunities for ERC students to live and work in other countries, broadening their knowledge and experience most relevant to success as an innovator in a global economy.
There were a few exemplary partnerships that did fulfill these goals. One of these was a partnership of the ERC for Revolutionizing Metallic Biomaterials (RMB) headquartered at NCAT and the University of Pittsburg. By the time of the proposed ERC’s site visit, they had formed a partnership with Frank Witte, an MD/Ph.D. Professor of Biomaterials Research and Regenerative Medicine at the University of Hannover Medical School in Germany. Dr. Witte has since moved to Berlin, where he serves as a Professor of Bioactive Implants at the Julius Wolff Institut and Berlin-Brandenburg Center for Regenerative Therapies, Charite– Universitaetsmedizin, Berlin, Germany.
At the time of the pre-award site visit in 2009, the RMB ERC was searching for increased experience with the use of the biodegradable material, magnesium, in humans. They found Prof. Witte, who had clinical expertise and experience in using biodegradable magnesium metallic alloys in animal and human trials. By the time of the start of the award, he had become an Adjunct Professor of Bioengineering, Swanson School of Engineering, Department of Bioengineering, University of Pittsburgh (Pitt) (effective January 1, 2009). Dr. Witte could then serve as the Ph.D. adviser for Pitt ERC graduate students. During the course of the award, Professor Witte became not only a dedicated member of the ERC but also a member of the broader ERC “family.” He attended several ERC Program Annual Meetings, contributing to discussions of how to develop effective partnerships with non-U.S. universities. One of the rewarding and yet challenging aspects of that partnership was an REU opportunity for RMB students to carry out research at the University of Hannover in Professor Witte’s laboratory. It was reported in an RMB newsletter as follows:
Professor Frank Witte has initiated and created a unique alliance together with the University of Pittsburgh, the University of Cincinnati and the North Carolina A&T State University. Professor Witte researches processes that enhance the fundamental knowledge and technology in the field of revolutionizing metallic biomaterials in order to improve biodegradable magnesium-based metal implants. As the Engineering Research Center-Revolutionizing Metallic Biomaterials (ERC-RMB) Global Site Coordinator, Professor Witte aims to create a global research environment in order to equip his students with the necessary global world vision, the latest technology know-how in the field and required leadership skills that will be needed when entering the work force.
This summer, five students from the University of Pittsburgh were in Hannover, Germany and performed their research at the laboratories of Hannover Medical School. Professor Witte’s program requires each student to develop research strategy and follow through with a research project that they present before exiting the program. A committee will then give constructive feedback and evaluate the students on their work and commitment to the program.[97]
Preston remembers discussions of this experience at one of the NCAT reviews, where Witte was enthusiastic to host the students but soon realized once they arrived that his laboratory had no experience with undergraduate students, who needed mentoring and supervision, and didn’t have a budget for materials. He found the resources he needed but advises others to anticipate that up-front. A means was created for ERC funds to support these REU students as they carried out their research overseas, per NSF regulations. Another issue related to the fact the REU students had to be “chaperoned” as they were young students. He hired someone to host the students in one of the dormitories and to accompany them when they went on excursions, and all went well.
7-D(e) Sustaining ERC Education Programs Post-graduation
As ERCs graduate from NSF ERC Program funding, it might be expected that programs not essential to their survival—i.e., everything other than research and industrial interaction—would be curtailed. A 2010 study found, surprisingly, that “…Most of the centers also continue to … conduct effective education programs (albeit with reduced educational outreach activities)…”98
The same study found that “Sixty-seven percent of the centers that had instituted precollege programs… continued them after graduation.”99 However, maintaining education programs after graduation always requires deliberate planning and effort, and there usually is some reduction in scope and scale of effort. An informal 2002 white paper described the challenges in maintaining education programs at their former strength.100 Strong leadership and advocacy on the part of the Center Director as well as the Education Director are usually the keys to success in this endeavor.
7-E Exemplars
Over three decades, the ERC Program has graduated thousands of engineers who were increasingly diverse and exceptionally capable of working across disciplines to advance knowledge and technology and serve as innovators in a global economy. These graduates have been guided in their efforts by teams of ERC faculty and the professional leaders of the ERC education programs. This section provides summaries of the achievements of just a few of these exemplary leaders and graduates.
7-E(a) Model ERC Educators
i. White House Mentoring Awardees
Martha Absher is the Associate Dean for Education and Outreach in the Pratt School of Engineering at Duke University. Starting in 1987, she was the Outreach Director for the Emerging Cardiovascular Technologies ERC at Duke and a strong contributor to the early evolution of ERC education programs, especially in diversity and inclusion. In 1996, Absher was one of the first 10 recipients of the Presidential Award for Excellence in Science, Mathematics, and Engineering Mentoring; she was honored for her mentoring of deaf students at Gallaudet University and African-American students at several HBCUs. See http://www.crpc.rice.edu/newsArchive/pres_award.html
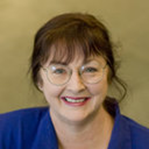
Martha Absher
Anne Donnelly is the Director of the Center for Undergraduate Research at the University of Florida. Beginning in 1995, she was the Education and Outreach Director of the Particle Engineering Research Center at UF, where she earned her Ph.D. in instruction and curriculum. During her time at PERC, Dr. Donnelly was one of the leading ERC Education Directors, contributing to the Education Programs chapter of the ERC Best Practices Manual and conducting a study of the sustainability of education programs in graduated ERCs. In 2013, she received the Presidential Award for Excellence in Science, Mathematics, and Engineering Mentoring for her work in the Southeast Alliance for Graduate Education and the Professoriate (an NSF-supported alliance known as SEAGEP). See http://paesmem.net/node/1262
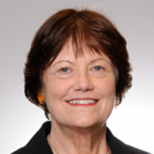
Anne Donnelly
Gary May was named the Chancellor of the University of California at Davis in 2017. He was Dean of Engineering at the Georgia Institute of Technology from July 2011 until June 2017. Earlier, while a member of Georgia Tech’s Electrical & Computer Engineering faculty and its microelectronics group, Dr. May had been the Education Director of the Packaging Research Center, an ERC at Georgia Tech. During that time, he founded Georgia Tech’s Summer Undergraduate Research in Engineering/Science (SURE) program, a summer research program designed to attract talented minority students into graduate school. He also founded and led the Facilitating Academic Careers in Engineering and Science program (FACES), designed to encourage minority engagement in engineering and science careers. In 2012, May received the Presidential Award for Excellence in Science, Mathematics, and Engineering Mentoring for these and other mentoring accomplishments. See https://en.wikipedia.org/wiki/Gary_S._May and https://www.nsf.gov/news/news_summ.jsp?cntn_id=134624&org=NSF&from=news
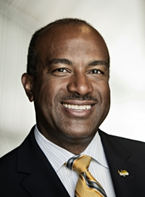
Gary May
ii. Education Scholar: Giselle Ragusa
Giselle Ragusa was an early leader of the ERC Program’s drive for formal assessment and evaluation of education programs in the ERCs. She was the Associate Director for Education and Outreach Assessment at the Biomimetic Microelectronic Systems (BMES) ERC, headquartered at the University of Southern California, and a professor in USC’s Viterbi School of Engineering’s Division of Engineering Education who specialized in STEM education. Dr. Ragusa promulgated a theoretical assessment approach based on how people learn. This approach was useful in familiarizing other ERCs’ Education Directors, at both pre-college and university education levels, with current theory bearing on their more outcome-oriented assessment approaches. Her work was partly responsible for the BMES “setting the standard for long-term pre-college partnerships” among ERCs.[98] Preston remembers that Ragusa’s horizons were expanded through the ERC experience as she had to learn how to integrate educational outcomes with ERC outcomes. She is now a Professor in the USC’s Viterbi School of Engineering’s Department of Engineering Education. See http://www-bcf.usc.edu/~ragusa/
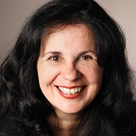
Gigi Ragusa
iii. University Education Leaders
Penny Jeffrey was the overall Education Program Director at the FREEDM Systems ERC at North Carolina State University. Her experiment with an ERC Graduate Portfolio of skills and knowledge required of all graduating ERC grad students is described in detail in Section 7-D(d). Jeffrey was a leader among the ERC Education Directors in developing center-wide education program strategies based on sound engineering education best practices. Today, Dr. Jeffrey is an Assistant Professor in the College of Education at NCSU. See https://ced.ncsu.edu/people/pmshumak/
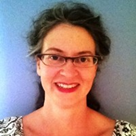
Penny Jeffrey
Leyla Conrad was, for six years starting in 1998, the Associate Director for Education and Outreach Programs of the Microsystems Packaging Research Center (PRC), at Georgia Tech. There, she developed and managed a comprehensive, multidisciplinary education program emphasizing globalization that established the model for microelectronics packaging education both nationally and internationally, targeting all levels from pre-college to professional practitioners. Concurrently, and continuing to the present, she has been the Outreach Director leading education and diversity programs for Georgia Tech’s School of Electrical & Computer Engineering, aiming to increase the number of incoming undergraduate students. Dr. Conrad is also Co-Director of the SURE Robotics program at Georgia Tech. Her BS, MS, and PhD degrees are all in Physics.
https://www.linkedin.com/in/leyla-conrad-3b201927/
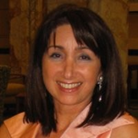
Leyla Conrad
Elizabeth Tranter was the Education and Outreach Director at the Center for Power Electronics Systems (CPES), based at Virginia Polytechnic Institute and State University (VPI). As the first ERC to have more than two affiliate institutions—it had five partners, including the University of Puerto Rico at Mayaguez—CPES presented complicated program management challenges that had not been addressed by earlier ERCs. These included coordinating the curriculum and graduation requirements of students from several different universities. Tranter broke new ground in accomplishing this, including building strong partnerships with minority-serving institutions. In 2015, she was appointed associate vice president for research planning in the Office of the Vice President for Research at VPI. Currently in semi-retirement, she serves as Grants Administrator for a large coastal county in South Carolina.
https://www.linkedin.com/in/elizabeth-tranter-2b13b860/
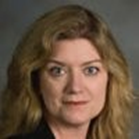
Beth Tranter
iv. Precollege Education Leaders
Claire Duggan has been the K-12 Outreach Director at the Bernard M. Gordon Center for Subsurface Sensing and Imaging Systems (CenSSIS), based at Northeastern University (NU), since its inception in 2000. Concurrently, she was Associate Director of the Center for the Enhancement of Science and Mathematics Education (CESAME) at NU. Her duties included overseeing coordination between CESAME and CenSSIS. Since 2008, she has also been the Director for Programs and Operations at NU’s Center for STEM (Science, Technology, Engineering and Mathematics) Education. Duggan is recognized nationally as a leader in the design and implementation of STEM educational outreach programs; within the ERC Program, she was a leader of the center pre-college education directors for the duration of CenSSIS. In 2015 Duggan was inducted into the Massachusetts Hall of Fame for Science Educators. Her work was greatly facilitated by the strong support for education programs at all levels on the part of the CenSSIS Center Director, Dr. Michael Silevitch. (See the linked file, “Claire Duggan on Leadership.”)
https://www.linkedin.com/in/cjduggan/
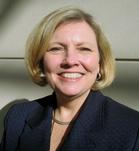
Claire Duggan
Joseph Cocozza was the Education & Outreach Director at USC’s Biomimetic Microelectronic Systems ERC. With a PhD in Biomedical Engineering, he had as a personal mission increasing the number of young students who are interested in pursuing science and engineering careers. For example, in 2007 he collaborated with BMES faculty and pre-college teachers to create four modules that taught fundamental science, math, and engineering principles in an age-appropriate manner and provided hands-on discovery experiences, using research conducted by researchers in the BMES laboratories as a starting point, for 3rd, 4th, and 5th grade students at a local elementary school in a program called Science for Life, and also for 500 middle-school students participating in a summer program at the Discovery Station Museum in Hagerstown, Maryland. Currently, Dr. Cocozza is an Assistant Professor of Research Ophthalmology in USC’s Keck School of Medicine.
https://profiles.sc-ctsi.org/joseph.cocozza
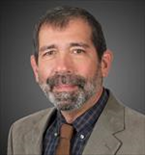
Joe Cocozza
Eddie Branchaud was co-president of the Neuromorphic Engineering Students’ Society at the Center for Neuromorphic Systems Engineering, an ERC at the California Institute of Technology. In 2002 on his own initiative he founded the Caltech Classroom Connection (CCC), which put Caltech graduate student volunteers in K-12 classrooms of the Pasadena Unified School District (PUSD) schools for three hours per week for a full semester. The Caltech students provided assistance answering science questions, helped with labs, and served as science and engineering role models in the classroom—key factors in enhancing a teacher’s ability bring the excitement of science and engineering concepts to the classroom. About 85% of the students in PUSD schools are from groups that are underrepresented in science and engineering. During its first two years alone, CCC partnerships between 12 Caltech students and 12 PUSD teachers impacted 600 students. After receiving his PhD in Mechanical Engineering at Caltech, Dr. Branchaud became a science teacher in the New York City Public Schools and at the LIFE School in Guatemala, where he was also Principal. Later he was Director of Operations at the Roxbury Prep Charter School in Boston; and currently he is a Principal Associate with Education Resource Strategies, a non-profit education consulting firm based in Watertown, MA.
https://www.erstrategies.org/people/eddie_branchaud
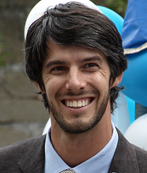
Eddie Branchaud
7-E(b) Exemplary ERC Graduates
Micro/Optoelectronics, Sensing, and IT Tech Cluster
Data Storage Systems Center (DSSC)–Class of 1990
Sally Doherty (PhD Electrical and Computer Engineering, Carnegie Mellon University, 1994-1998) is Vice President and General Manager of the Preamp Components Division of Broadcom. In this role, Doherty leads the business unit responsible for developing analog and mixed-signal products and technologies for storage markets. Dr. Doherty joined Broadcom through their acquisition of LSI Corporation, where she served as Vice President of Research and Development for the Storage Peripherals Division. There, she was responsible for product development and led the LSI global expansion strategy in China. Before joining LSI, Dr. Doherty worked at Lucent Technologies and its spinoff, Agere Systems, in a variety of engineering and management roles.
https://www.linkedin.com/in/sallydohertyminnesota/
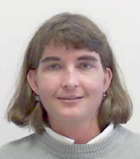
Sally Doherty as a student
Integrated Media Systems Center (IMSC)–Class of 1996
Ali Dashti (MS Computer Engineering, PhD Computer Science, USC, 1993-1999) is currently the Chairman of Kuwait and Gulf Link Holding Company (KGL Holding), Vice Chairman and Chief Executive Officer at KGL Logistics, and Vice Chairman at Kuwait and Gulf Link Transport Company (KGL). Over the past 20 years, Dashti has served in many leadership positions in the transportation and logistics industry, in which he founded several spinoff companies, including E-Learning Company and Axis Solutions (an IT System Integrator specialized in transportation solutions). During his tenure at KGL Holding, Dr. Dashti has been instrumental in expanding KGL’s business with U.S. Government by more than $2 billion, and the company is now one of the premier logistics providers to the U.S. Armed Forces in the Middle East. Prior to his graduation from USC, he served as a Ph.D. Graduate Student Research Assistant (under the advisement of Prof. Cyrus Shahabi) in the IMSC, where he participated in the design and implementation of numerous research projects. He also served as the first President of IMSC’s Student Leadership Council. He later obtained an MBA from the University of Chicago. In 2003, Dr. Dashti published a book entitled Streaming Media Servers with his colleagues at USC.
https://www.linkedin.com/in/ali-dashti-26a61232/
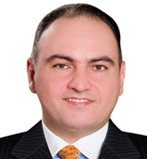
Ali Dashti
Center for Integrated Access Networks (CIAN)–Class of 2008
Brittany Lynn (PhD in Optical Sciences, University of Arizona, 2010-2015) is a rising U. S. Navy laboratory scientist and subject matter expert in Ultra-Short Pulse Laser-induced filamentation for modeling, experimentation, and applications at the Space and Naval Warfare Systems Center–Pacific. Lynn currently directs projects ranging from basic plasma physics and laser filamentation applications to free-space underwater communications systems. Her work in next-generation optical propagation modeling not only supports the development of these technologies but has the potential to be implemented as an operational tool across the DoD Directed Energy portfolio. In 2017, Dr. Lynn was awarded the three-year OSD(R&E) Laboratory University Collaboration Initiative Fellowship to probe the ability of orbital-angular-momentum imparted on the laser beam to enable further control of the laser filamentation process.
https://www.linkedin.com/in/brittany-lynn-10b3a243/
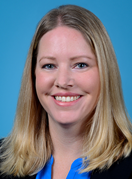
Brittany Lynn
Advanced Manufacturing Tech Cluster
Reconfigurable Manufacturing Systems (RMS) ERC–Class of 1996
Burak Ozdoganlar (PhD Mechanical Engineering, 1996-1999, University of Michigan) is the Ver Planck Endowed Chair Professor of Mechanical Engineering, Biomedical Engineering, and Materials Science & Engineering at Carnegie Mellon University (CMU). He is also the Associate Director of the Engineering Research Accelerator and Director of the Multiscale Manufacturing and Dynamics Laboratory at CMU. Dr. Ozdoganlar was the first President (1997-1999) of the Student Leadership Council at the RMS, which was one of the first ERC SLCs. In that role, he organized and led an annual SWOT analysis of the ERC to obtain student perspectives on their ERC involvement—one of the first of what became an ERC Program-wide requirement. He also chaired the working group of students who wrote the ERC Best Practices manual chapter on Student Leadership Councils.
https://www.linkedin.com/in/burak-ozdoganlar-a323b214/
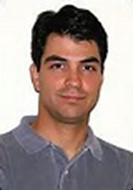
Burak Ozdoganlar
The Statistics of Manufacturing Translate to the Statistics of Money Min Zhang came to the University of Michigan in 2002 from China, where she had earned her B.S. and M.S. in Mechanical Engineering, to pursue her doctoral degree. At Michigan, she joined the Reconfigurable Manufacturing Systems ERC, led by Center Director Yoram Koren. Her PhD advisor was Prof. Jun Ni, who is an expert on statistics in manufacturing processes. Soon after taking her PhD in ME with an emphasis on statistics, in 2006, Min was one of approximately 400 applicants who were interviewed in Chicago for a position with the Bank of America. More than 80% of the interviewees were graduates of a business school; a few also had an engineering background. After two days of interviews, the pool of applicants was narrowed down to 50 candidates, and their interviews continued; Min was among the 50. Having seen so many highly qualified candidates, she was actually surprised to be a finalist and asked the person who contacted her, “Are you sure that I’m among the remaining 50? I’ve been a student—I don’t know much about money!” The answer was: “Yes, but you know statistics very well. Only a few of the people from business school know statistics, and that’s what we need.” Ten out of the 400 were hired. Min was one of them. Twelve years later, Dr. Min Zhang is a Senior Quantitative Finance Manager for the Bank of America. She is a Director in Global Risk Analytics, leading an econometric modeling team of 25 people. Dr. Zhang says, “This year (2018) I was selected to go through an Accelerated Leader Experience program that will enable me to achieve the next level of my career, hopefully in the near future. I credit both Prof. Ni and Dr. Koren for all their support while I was a student at Michigan. I feel very proud about my solid engineering background, which lays the foundation for the quantitative space I am leading right now.” |
Min Zhang (PhD Mechanical Engineering/Statistics, University of Michigan, 2002-2006) is a Senior Quantitative Finance Manager for the Bank of America and a Director in Global Risk Analytics. She earned her doctoral degree through the Reconfigurable Manufacturing Systems ERC. Zhang began with the Bank of America in 2007 as a Quantitative Analyst and moved up the career ladder as a Quantitative Finance Manager, specializing in econometric modeling.
https://www.linkedin.com/in/min-zhang-0548758/
Center for Biorenewable Chemicals (CBiRC)–Class of 2008
Alexis Campbell (PhD in Biochemistry, Biophysics & Molecular Biology, Iowa State University, 2011) is the Director of Iowa State’s Science Bound program, the University’s pre-college program to empower Iowa students of color to pursue degrees and careers in STEM fields. She began her graduate work as a George Washington Carver Fellow, which was followed quickly by the Promising Young Scientist Award at Iowa’s NAACP Black Hall of Fame Banquet. During her final year as a doctoral student she was awarded an NSF-GK12 Fellowship through Iowa State’s Symbi GK-12 Program. This experience changed the trajectory of Campbell’s career, as it empowered her to develop a new CBiRC-inspired laboratory module at the interface between research and education. This module put into undergraduate teaching laboratory classes research-based, hypothesis-driven laboratory exercises that teach fundamental concepts in molecular genetics, biochemistry, and bioengineering. Dr. Campbell’s effort was recognized by further NSF support that allowed her to disseminate the module nationwide to the University of Illinois at Urbana-Champaign, Ohio State University, the University of California-Merced, and the University of Texas.
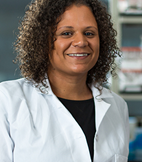
Alexis Campbell
Biotechnology and Health Care Tech Cluster
Biotechnology Process Engineering Center (BPEC)–Class of 1985Noubar Afeyan(PhD Biochemical Engineering, 1987, MIT) is a biotechnology entrepreneur, venture capitalist, inventor, technologist, and CEO. In 1988, shortly after obtaining his PhD, Afeyan founded PerSeptive Biosystems. While CEO of PerSeptive, heco-founded and funded numerous other biotechnology companies, including: ChemGenics Pharmaceuticals, acquired by Millennium Pharmaceuticals in 1997; Exact Sciences;Agenus; and Color Kinetics, which was later acquired by Philips. After PerSeptive’s acquisition by Perkin Elmer/Applera Corporation in 1998, Afeyanbecame Senior Vice President and Chief Business Officer of Applera, where he initiated and oversaw the creation of Celera Genomics.In 2000, Afeyan founded Flagship Pioneering to create and fund early-stage start-ups addressing unmet needs in healthcare and sustainability. Among a number of other companies which Afeyan has founded or helped start is Moderna Therapeutics(now Moderna Inc.), which at the time of writing (May 2020) is attempting to develop a vaccinebased on mRNA therapeuticsto combat the SARS-CoV-2 coronavirus. He is a Senior Lecturer at the MIT Sloan School of Management. https://www.linkedin.com/in/noubarafeyan/
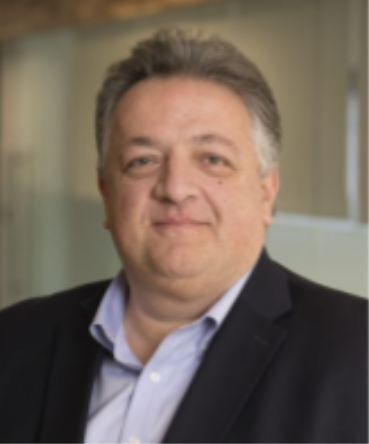
Noubar Afeyan
Engineered Biomaterials Engineering Research Center (UWEB)–Class of 1996
Michael Garrison (PhD Bioengineering, 1995-1999, University of Washington) is the worldwide leader of R&D for the Becton Dickinson (BD) Medication and Procedural Solutions Business Unit. Dr. Garrison is accountable for all aspects of Strategic Innovation, Technology Development, Portfolio Execution and Delivery, and Product Engineering at BD, and is responsible for talent development and management of a global team of over 400 people and an annual budget of over $100 million. When he first joined BD in 2005 as Director of Advanced Technology, Dr. Garrison led a change management effort that resulted in implementation of improved global product, technology, and concept development processes for the company. Prior to BD, Dr. Garrison served as Manager of R&D for the surgical heart valve franchise at Edwards Lifesciences, Global Marketing Manager at Roche Diagnostics, and as a Research Scientist at Cygnus Therapeutic Systems. In these roles he was responsible for developing and commercializing new medical technologies in global markets.
https://www.linkedin.com/in/michael-garrison-b7953117/
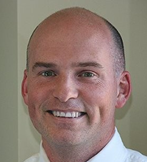
Michael Garrison
Center for the Engineering of Living Tissues (GTEC)–Class of 1998
Manu Platt (PhD Biological Engineering, 2002-2006, Georgia Tech) is an Associate Professor of Biomedical Engineering at Georgia Tech. As an undergraduate at Atlanta’s Morehouse College he was invited to carry out research at the joint Georgia Tech/Emory Center for the Engineering of Living Tissues (GTEC), an ERC, as a part of the GTEC team. This experience changed his career path and he decided to pursue a Ph.D. in biological engineering at Georgia Tech. After completing his doctoral degree, he was employed as a postdoctoral fellow in Dr. Linda Griffith’s laboratory at MIT’s Biotechnology Process Engineering Center, a graduated ERC. In 2009, Dr. Platt returned to Georgia Tech to join the Biomedical Engineering faculty, where he is affiliated with GTEC. The focus of his research is on tissue remodeling, repair, and regeneration. In 2010 he received a $1.5 million NIH Director’s New Innovator Award to support a project aimed at reducing the incidence of stroke in children with sickle cell disease. Through his Platt Lab, Platt is very active in mentoring the next generation of biomedical engineers and increasing their diversity. He is currently the Pre-college Education Director for the ERC for Cell Manufacturing Technologies (CMaT) at Georgia Tech.
https://platt.gatech.edu/index.html
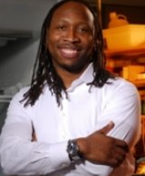
Manu Platt
Biomimetic MicroElectronic Systems (BMES) ERC–Class of 2003
Noelle Stiles (PhD Computation and Neural Systems, 2009-2014, Caltech) was mentored while still in high school by a faculty member of the BMES ERC at USC and won awards for her science fair projects. She is currently a postdoctoral fellow at the University of Southern California’s Keck School of Medicine, where she focuses on the study of multimodal interactions among the various senses. Her graduate research at Caltech focused on the design and evaluation of sensory substitution devices for rehabilitation of the blind.
http://www.its.caltech.edu/~nstiles/about.htm
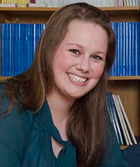
Noelle Stiles
CASE STUDY When Noelle Stiles was an 11th-grader in Fall 2003, she began working with BMES Prof. Armand R. Tanguay, Jr., after she was introduced to him at a science fair. Initially, Tanguay mentored her science fair project, and then accepted her as a full member of his Optical Materials and Devices Laboratory at USC when it became apparent that she was deeply committed to her research. As a full member of the lab, Noelle participated in the Retinal Prosthesis Research Group at USC’s Keck School of Medicine, where she attended an FDA surgical trial of a retinal prosthetic microelectrode and participated intensively in the successful surgical implantation of the first intraocular camera in a dog’s eye. In the 2004 Orange County Science & Engineering Fair, her project, “Intraocular Camera for Retinal Prostheses: Restoring Vision to the Blind,” took the Grand Sweepstakes Award, Senior Division (the top prize of the entire Fair). That same year she was named one of 300 semifinalists in the Siemens Westinghouse Competition in Math, Science, and Technology at the national level. Entering USC as an undergraduate, Noelle continued her research within the BMES ERC on the visual psychophysics and optical systems design criteria applicable to various camera designs for retinal prosthetic devices. Less than three years after discovering her passion for biomedical research as a high school junior, this remarkable young student was routinely presenting her research at professional conferences, where she was accepted as a peer. |
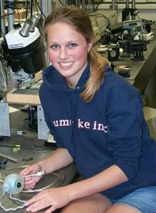
Noelle Stiles as a high school student researcher in the lab at USC’s BMES ERC |
ERC for Revolutionizing Metallic Biomaterials (RMB)–Class of 2008
Leon White (PhD Mechanical Engineering, 2010-2014, North Carolina Agricultural and Technical State University (NCAT)) is an Operations Program Manager at Northrup Grumman Corporation, where he serves as the primary interface between the program office and manufacturing. While a student at NCAT, White served as the President of the RMB’s Student Leadership Council and as the student representative to its Education Advisory Board. Dr. White’s research at the RMB focused on coatings to inhibit the corrosion of magnesium and its alloys for for possible biomedical applications.
https://www.linkedin.com/in/lgwhite
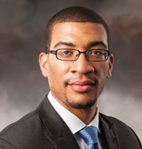
Leon White
Energy, Sustainability, and Infrastructure Tech Cluster
Center for Advanced Technology for Large Structural Systems (ATLSS) ERC–Class of 1986
Maria Garlock (B.S., Civil Engineering, 1991, and Ph.D., Structural Engineering, 2003, Lehigh University) is a Professor of Civil and Environmental Engineering at Princeton University. As a Latina student, she was an REU student at ATLSS during the time of the ERC award. Garlock has been recognized at Princeton for both her teaching and research. In 2012 she received the President’s Award for Distinguished Teaching, which is given to four faculty for their record of distinguished teaching at Princeton. In 2016 she received the T.R. Higgins Lectureship Award, which recognizes an outstanding lecturer and author whose technical papers are considered an outstanding contribution to the engineering literature on fabricated structural steel.
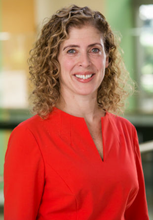
Maria Garlock
Robert Fleischman (Ph.D., Civil Engineering, 1989-1995) is a Professor in the Department of Civil Engineering and Engineering Mechanics at the University of Arizona. He received both his M.S. and Ph.D. degrees at ATLSS during the time of its ERC Program funding. Specializing in Structures, Fleischman spent three years as a supervising engineering in the construction industry before entering academe.
http://civil.arizona.edu/robert-fleischman
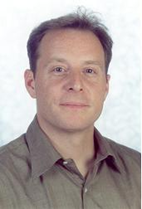
Robert Fleischman
Future Renewable Electric Energy Delivery and Management (FREEDM) Systems Center–Class of 2008
Babak Parkhideh (Ph.D., Electrical Engineering, 2008-2012, North Carolina State University) is an Associate Professor in the Electrical and Computer Engineering Department at the University of North Carolina at Charlotte and the Founding Director of the PV Integration Laboratory there. He specializes in Power Electronics and Power Electronics Applications. Parkhideh was previously Director of Power Electronics and Systems at Sinewatt Inc., and is currently President of a startup, Tell-I Technologies.
https://www.linkedin.com/in/babak-parkhideh-77702111/
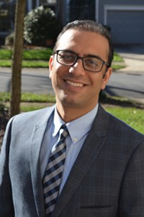
Babak Parkhideh
[1] National Academy of Engineering (1984). Guidelines for Engineering Research Centers: A Report to the National Science Foundation. Washington, DC: National Academy Press, p. ii. [https://doi.org/10.17226/19472]
[2] National Research Council (1986). The New Engineering Research Centers—Purposes, Goals, and Expectations. Report of the Cross-Disciplinary Research Committee. National Research Council. Washington, DC: National Academy Press. p. 5. [https://doi.org/10.17226/616]
[3] National Academy of Engineering (1984), op. cit., p. 3.
[4] Ibid., p. 6.
[5] Ibid., p. 5.
[6] Ibid., p. 1.
[7] Churchman, C. West (1979). The Systems Approach. New York: Laurel/Dell Publishing Co., Inc.
[8] NSF (1984). Op. cit., p. 1.
[9] NSF (1984). Op. cit., p. 1.
[10] National Research Council (1986). Report to the National Science Foundation Regarding the Systems Aspects of Cross Disciplinary Engineering Research. Cross-Disciplinary Engineering Research Committee. Washington, DC: National Academy Press. [https://doi.org/10.17226/19220]
[11] Ibid., p. 2.
[12] Ibid., p. 1
[13] Ibid., p. 2.
[14] NSF (1995). Highlights of Engineering Research Centers Education Programs (NSF 95-56). Arlington, VA: National Science Foundation, p. 4.
[15] BPEC was the only ERC ever to successfully recompete and complete its full second 10-year term as an ERC.
[16] National Research Council (1987). The Engineering Research Centers: Leaders in Change. Cross-Disciplinary Engineering Research Committee, summary of a symposium. Washington, D.C.: National Academy Press, p. 98. [https://doi.org/10.17226/18889]
[17] Later renamed the Institute for Systems Research.
[18] NSF (1995). Highlights, op. cit., p. 34.
[19] National Research Council (1987), op. cit., pp. 56-57.
[20] NSF (1995), Highlights, op. cit., p. 31.
[21] Ibid., p. 45.
[22] Ibid., pp. 26-28.
[23] National Research Council (1987), op. cit., pp. 46-47.
[24] Ibid., p. 99.
[25] Ibid., pp. 92-93.
[26] NSF (1995), op. cit., p. 28.
[27] Costerton, J. William (1996). The ERC Model for a “New PhD.” White Paper published by the Center for Biofilm Engineering, Montana State University, Bozeman, MT.
[28] Ibid.., pp. 33-34.
[29] Ibid., pp. 32-40.
[30] Engineering Centers Division (1991). The ERCs: A Partnership for Competitiveness, Report of a Symposium, February 28-March 1, 1990. Washington, DC: Directorate for Engineering. National Science Foundation. NSF 91-9. p. 17.
[31] Ibid.
[32] Ibid, p. 18.
[33] Ibid., pp.18-21.
[34] Fitzsimmons, Stephen J., Oren Grad, Bhavya Lal, and Ken Carlson (1996). Job Performance of Graduate Engineers who Participated in the NSF Engineering Research Centers (ERC) Program. Cambridge, MA: Abt Associates, NSF Contract 94-131561(3), September 1996. p. iii.
[35] Ibid., pp iii-iv.
[36] Ibid., p. 72.
[37] Ibid., p. 76.
[38] Ibid., Appendix C., question 30 response.
[39]Roessner, J. David, David W. Cheney, H.R. Coward (2004). Impact on Industry of Interactions with Engineering Research Centers – Repeat Study, Summary Report. SRI International (P11537), December 2004, p. 28.
[40] NSF (1997). Program Solicitation, Engineering Research Centers, Partnerships with Industry and Academe for Next-Generation Advances in Research, Technology, and Education. Fiscal Year 1997. Directorate for Engineering, National Science Foundation, NSF 97-5, p. 2.
[41] https://pratt.duke.edu/about/news/reu-25-positive-impact-students%E2%80%94and-duke-engineering
[42] CESAME was initially supported as an experimental Industry/University Cooperative Research Center focused on pre-college engineering education, and later by NSF’s education programs, and the State of Massachusetts. https://www.nsf.gov/awardsearch/showAward?AWD_ID=9203420&HistoricalAwards=false
[43] ERC Best Practices Manual (2002). Chapter 4, Education and Outreach, pp. 4-37–4-38.
[44] Ibid., p. 4-67.
[45] See Cady, Elizabeth and Norma Fortenberry (2008). Content Analysis of The History 0f NSF Funding for Engineering Education Research (Ac 2008-159). Washington. DC: National Academy of Engineering, for a broader history of NSF’s support of engineering education.
[46] https://ec.poole.ncsu.edu/undergraduate-programs/tec-certificate/
[47] NSF (1998). Engineering Research Center for Bioengineering Educational Technology. Program Announcement NSF 98-68. .
[48] Preston, Lynn (2007). Education in Centers 1985–2007. Briefing to the National Science Board in Open Session, Slide 15.
[49] ERC Best Practices Manual (2002). Chapter 4, Education and Outreach, pp. 4-65.
[50] Howard, Larry (2003). Adaptive learning technologies in bioengineering education. IEEE Engineering in Medicine and Biology Magazine, July/August 2003. Pp. 58-65.
[51] ERC Best Practices Manual (2002), op. cit.
[52] Personal communication to Lynn Preston, September 1, 2019.
[53] The use of these insights, drawn from the ERC’s final report, was approved by Dr. Harris in August 2019.
[54] Initially some of these staff were titled Education Coordinator. As the importance of the position became recognized and its responsibilities grew, the title Education Director (or often, Education and Outreach Director) became the norm. At a number of centers, the Coordinator position remained in a subordinate role under the Education Director, who often had a PhD and in many cases was a faculty member.
[55] NSF (1994). Engineering Research Centers. Program Announcement Fiscal Year 1994. Directorate for Engineering, National Science Foundation, NSF 94-150, p. 2.
[56] ERC Best Practices Manual, Ch. 4, “Education Programs.” <http://erc-assoc.org/content/chapter-4-education-programs>
[57] ERC Best Practices Manual (2002), op. cit., pp. 4-10 – 4-11
[58] Ibid., p. 4-12.
[59] Ibid., pp. 4-20 – 4-21.
[60]Ibid., pp. 4-45 – 4-46.
[61] Ibid., pp. 4-46 – 4-47.
[62] Ibid., p. 4-47
[63] NSF (1995). Op. cit., pp. 33-34.
[64] ERC Best Practices Manual (2002), op. cit., p. 4-45.
[65] Ibid., p. 4-52.
[66] Ibid., p. 4-35.
[67] Ibid., p. 4-52.
[68] Ailes, Catherine P., Irwin Feller, and H. Roberts Coward. (2001). The Impact of Engineering Research Centers on Institutional and Cultural Change in Participating Universities, Final Report. Stanford Research Institute. Arlington, VA. Stanford Research Institute. p. 112. http://erc-assoc.org/sites/default/files/studies_reports/ERC%20Cultural%20Impact_SRI%202001.pdf
[69] NSF (1995). Highlights, op. cit., pp. 8-10.
[70] Ailes et al., op. cit., pg. 44. Preston remembers finding out from site visits to the Mississippi State ERC that some of the graduates from this electronic visual imaging program, i.e., computer graphics, migrated to Hollywood to form the first cadre of technicians developing animated films by digital means.
[71] Ailes et al., op. cit., pp. 112-113.
[72] Ibid., pp. 113-114.
[73] Ibid., p. 115.
[74] Ibid., p. 117.
[75] ERC Best Practices (2002), op. cit., pp. 4-7 – 4-8.
[76] NSF (2001). “Pre-College Outreach Programs in ERCs and EERCs, FY 1998-2001: Highlights.” Internal ERC Program compilation, March 2001.
[77] National Science Foundation (2002). Program Solicitation, Engineering Research Centers, Partnerships in Transforming Research, Education and Technology Fiscal Year 2002. NSF 02-24. p. 7.
[78] National Science Foundation (2007). Moving Forward to Improve Engineering Education, National Science Board, November 19, 2007 (NSB-07-122)
[79] Poats, Mary (2007). Directorate for Engineering Research Experiences for Teachers (RET) Program. Report prepared for NSF internal use.
[80] Soyster, Allen L. Education Programs of the Engineering Research Centers: Building Future Leaders in Engineering Innovation. Gordon Prize Nomination, April 2008.
[81] http://2018.igem.org/Main_Page
[82] National Science Foundation (2007). Engineering Research Centers (ERC): Partnerships in Transforming Research, Education, and Technology. FY 2007 Program Solicitation. NSF 07-521, p. 7. < https://www.nsf.gov/pubs/2007/nsf07521/nsf07521.htm>
[83] The bold text indicates new, additional Gen-3 features.
[84] ERC Best Practices Manual, Chapter 4, Education Programs, op. cit., Appendix 4.5. http://erc-assoc.org/content/appendix-45-graduate-education-examples
[85] Ibid.
[86] NCAT, the RMB ERC, the ERC Program, and the Nation owe a debt of gratitude to Professor Devdas Pai, the RMB’s Education, Outreach and Diversity Director from NCAT, who devoted so many hours to developing the new degree programs and seeing them through the legislative and ABET approval processes—one of the later achievements of his career at NCAT, as he died in 2017.
[87] http://hibp.ecse.rpi.edu/~connor/Illumineer/
[88] http://erc-assoc.org/content/appendix-43-precollege-and-community-engagement-examples (4.3.3.2)
[89] Ibid., 4.3.2.3
[90] Ibid, 4.3.3.1
[91] Ibid., 4.3.7.2
[92] Engineering Education and Centers Division (2008). Designing the Next-Generation ERC: Key Features, Report from the 2007 Annual Meeting of The Engineering Research Centers Program. Arlington, VA; National Science Foundation. January 2008, pp. 6-7.
[93] You can watch the remarks of Dr. Peterson about the ERCs, the Perfect Pitch Contest, and his time at NSF at https://www.youtube.com/watch?v=wNZHx9gycUk
[94] You can watch Sebastian Husein’s presentation on https://www.youtube.com/watch?v=9vFR0Z0UaG8
[95] QoLT Briefing to Congress, hosted by the American Chemical Society, Slide 7. February 9, 2012.
[96] https://www.cmu.edu/piper/news/archives/2011/september/qoltfoundry.html
[97] http://erc.ncat.edu/?article=151
[98] Preston, Lynn (2012). “Engineering Research Centers: Transformational Partnerships in Research, Education, and Innovation,” 2012 ERC annual meeting presentation, slide 18.